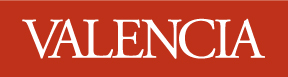
BSC 1011C
General Biology II
Dr.
Graeme Lindbeck
glindbeck@valenciacollege.edu
The Origins of Eukaryotic Diversity
Outline
A. Introduction to the Protists
- Systematists have split protists into many kingdoms
- Protists are the most diverse of all eukaryotes
B. The Origin and Early Diversification of Eukaryotes
- Endomembranes contributed to larger, more complex cells
- Mitochondria and plastids evolved from endosymbiotic bacteria
- The eukaryotic cell is a chimera of prokaryote ancestors
- Secondary endosymbiosis increased the diversity of algae
- Research on the relationships between the three domains is changing ideas about
the deepest branching in the tree of life
- The origin of eukaryotes catalyzed a second great wave of diversification
C. A Sample of Protistan Diversity
- Diplomonadida and Parabasala: Diplomonads and parabasilids lack mitochondria
- Euglenozoa: The euglenozoa includes both photosynthetic and heterotrophic flagellates
- Alveolata: The alveolates are unicellular protists with subsurface cavities
(alveoli)
- Stramenopila: The stramenopile clade that includes the water molds and heterokont
algae
- Structural and biochemical adaptations help seaweeds survive and reproduce
at the ocean's margins
- Some algae have life cycles with alternating multicellular haploid and diploid
generations
- Rhodophyta: Red algae lack flagella
- Chlorophyta: Green algae and plants evolved from a common photoautotrophic
ancestor
- A diversity of protists use pseudopodia for movement and feeding
- Mycetozoa: Slime molds have structural adaptations and life cycles that enhance
their ecological roles as decomposers
- Multicellularity originated independently many times
Introduction
Protists are eukaryotes and thus are much more complex than the prokaryotes.
The first eukaryotes were unicellular.
- Not only were they the predecessor to the great variety of modern protists, but
also to all other eukaryotes - plants, fungi, and animals.
The origin of the eukaryotic cell and the emergence of multicellularity unfolded
during the evolution of protists.
Eukaryotic fossils date back 2.1 billion years and "chemical signatures"
of eukaryotes date back 2.7 billion years.
For about 2 billion years, eukaryotes consisted of mostly microscopic organisms
known by the informal name "protists."
1. Systematists have split protists into many kingdoms
In the five-kingdom system of classification, the eukaryotes were distributed
among four kingdoms: Protista, Plantae, Fungi, and Animalia.
- The plant, fungus, and animal kingdoms are surviving the taxonomic remodeling
so far, though their boundaries have been expanded to include certain groups formerly
classified as protists.
- However, systematists have split protists into many kingdoms.
- Modern systematists has crumbled the former kingdom of protists beyond repair.
Protista was defined partly by structural level (mostly unicellular eukaryotes)
and partly by exclusion from the definitions of plants, fungi, or animals.
However, this created a group ranging from single-celled microscopic members,
simple multicellular forms, and complex giants like seaweeds.
The kingdom Protista formed a paraphyletic group, with some members more closely
related to animals, plants, or fungi than to other protists.
Systematists have split the former kingdom Protista into as many as 20 separate
kingdoms.
Still,"protist" is used as an informal term for this great diversity
of eukaryotic kingdoms.
Back to top
2. Protists are the most diverse of all eukaryotes
Protists are so diverse that few general characteristics can be cited without
exceptions.
Most of the 60,000 known protists are unicellular, but some are colonial and others
multicellular.
While unicellular protists would seem to be the simplest eukaryotic organisms,
at the cellular level they are the most elaborate of all cells.
- A single cell must perform all the basic functions performed by the collective
of specialized cells in plants and animals.
Protists are the most nutritionally diverse of all eukaryotes.
- Most protists are aerobic, with mitochondria for cellular respiration.
- Some protists are photoautotrophs with chloroplasts.
- Still others are heterotrophs that absorb organic molecules or ingest larger food
particles.
- A few are mixotrophs, combining photosynthesis and heterotrophic nutrition.
Euglena, a single celled mixotrophic protist, can use chloroplasts to
undergo photosynthesis if light is available or live as a heterotroph by absorbing
organic nutrients from the environment.
These various modes of nutrition are scattered throughout the protists.
- The same group may include photosynthetic species, heterotrophic species, and
mixotrophs.
While nutrition is not a reliable taxonomic characteristic, it is useful
in understanding the adaptations of protists and the roles that they play in
biological communities.
- Protists can be divided into three ecological categories:
- protozoa - ingestive, animal-like protists
- absorptive, fungus-like protists
- algae - photosynthetic, plant-like protists.
Most protists move with flagella or cilia during some time in their life cycles.
The eukaryotic flagella are not homologous to those of prokaryotes.
- The eukaryotic flagella are extensions of the cytoplasm with a support of the
9 + 2 microtubule system.
- Cilia are shorter and more numerous than flagella.
- Cilia and flagella move the cell with rhythmic power strokes, analogous to the
oars of a boat.
Reproduction and life cycles are highly varied among protists.
Mitosis occurs in almost all protists, but there are many variations in the process.
Some protists are exclusively asexual or at least employ meiosis and syngamy (the
union of two gametes), thereby shuffling genes between two individuals.
Others are primarily asexual but can also reproduce sexually at least occasionally.
Protists show the three basic types of sexual life cycles, with some other variants,
too.
The haploid stage is the vegetative stage of most protists, with the zygote as
the only diploid cell.
Many protists form resistant cells (cysts) that can survive harsh conditions.
Protists are found almost anywhere there is water.
- This includes oceans, ponds, and lakes, but also damp soil, leaf litter, and other
moist terrestrial habitats.
- In aquatic habitats, protists may be bottom-dwellers attached to rocks and other
anchorages or creeping through sand and silt.
- Protists are also important parts of the plankton, communities of organisms
that drift passively or swim weakly in the water.
- Phytoplankton (including planktonic eukaryotic algae and prokaryotic
cyanobacteria) are the bases of most marine and freshwater food chains.
Many protists are symbionts that inhabit the body fluids, tissues, or cells of
hosts.
These symbiotic relationships span the continuum from mutualism to parasitism.
- Some parasitic protists are important pathogens of animals, including those that
cause potentially fatal diseases in humans.
Back to top
B. The Origin and Early Diversification of Eukaryotes
The evolution of the eukaryotic cell led to the development of several unique
cellular structures and processes.
These include:
- membrane-enclosed nucleus,
- the endomembrane system,
- mitochondria,
- chloroplasts,
- the cytoskeleton,
- 9 + 2 flagella,
- multiple chromosomes of linear DNA with organizing proteins, and
- life cycles with mitosis, meiosis, and sex.
1. Endomembranes contributed to larger, more complex cells
The small size and simple construction of a prokaryotes imposes limits on the
number of different metabolic activities that can be accomplished at one time.
- The relatively small size of the prokaryote genome limits the number of genes
coding for enzymes that control these activities.
- In spite of this, prokaryotes have been evolving and adapting since the dawn of
life, and they are the most widespread organisms even today.
One trend was the evolution of multicellular prokaryotes, where cells specialized
for different functions.
A second trend was the evolution of complex communities of prokaryotes, with species
benefiting from the metabolic specialties of others.
A third trend was the compartmentalization of different functions within single
cells, an evolutionary solution that contributed to the origins of eukaryotes.
Under one evolutionary scenario, the endomembrane system of eukaryotes (nuclear
envelope, endoplasmic reticulum, Golgi apparatus, and related structures) may
have evolved from infoldings of plasma membrane.
Another process, called endosymbiosis, probably led to mitochondria, plastids,
and perhaps other eukaryotic features.
Back to top
2. Mitochondria and plastids evolved from endosymbiotic bacteria
The evidence is now overwhelming that the eukaryotic cell originated from a
symbiotic coalition of multiple prokaryotic ancestors.
A mechanism for this was originated by a Russian biologist C. Mereschkovsky and
developed extensively by Lynn Margulis of the University of Massachusetts.
The theory of serial endosymbiosis proposes that mitochondria and chloroplasts
were formerly small prokaryotes living within larger cells.
- Cells that live within other cells are called endosymbionts.
The proposed ancestors of mitochondria were aerobic heterotrophic prokaryotes.
The proposed ancestors of chloroplasts were photosynthetic prokaryotes.
These ancestors probably entered the host cells as undigested prey or internal
parasites.
- This process would be facilitated by the presence of an endomembrane system and
cytoskeleton, allowing the larger host cell to engulf the smaller prokaryote and
to package them within vesicles.
This evolved into a mutually beneficial symbiosis.
- A heterotrophic host could derive nourishment from photosynthetic endosymbionts.
- In an increasingly aerobic world, an anaerobic host cell would benefit from aerobic
endosymbionts that could exploit oxygen.
As host and endosymbiont evolved, both would become more interdependent, evolving
into a single organism, its parts inseparable.
- All eukaryotes have mitochondria or genetic remnants of mitochondria.
- However, not all eukaryotes have chloroplasts.
The serial endosymbiosis theory supposes that mitochondria evolved before chloroplasts.
Many examples of symbiotic relationships among modern organisms are analogous
to proposed early stages of the serial endosymbiotic theory.
Several lines of evidence support a close similarity between bacteria and the
chloroplasts and mitochondria of eukaryotes.
- These organelles and bacteria are similar is size.
- Enzymes and transport systems in the inner membranes of chloroplasts and mitochondria
resemble those in the plasma membrane of modern prokaryotes.
- Replication by mitochondria and chloroplasts resembles binary fission in bacteria.
- The single circular DNA in chloroplasts and mitochondria lack histones and other
proteins, as in most prokaryotes.
- Both organelles have transfer RNAs, ribosomes, and other molecules for transcription
of their DNA and translation of mRNA into proteins.
- The ribosomes of both chloroplasts and mitochondria are more similar to those
of prokaryotes than to those in the eukaryotic cytoplasm that translate nuclear
genes.
A comprehensive theory for the origin of the eukaryotic cell must also account
for the evolution of the cytoskeleton and the 9 + 2 microtubule apparatus of the
eukaryotic cilia and flagella.
- Some researchers have proposed that cilia and flagella evolved from symbiotic
bacteria (especially spirochetes).
- However, the evidence for this proposal is weak.
Related to the evolution of the eukaryotic flagellum is the origin of mitosis
and meiosis, processes unique to eukaryotes that also employ microtublules.
- Mitosis made it possible to reproduce the large genomes in the eukaryotic nucleus.
- Meiosis became an essential process in eukaryotic sex.
Back to top
3. The eukaryotic cell is a chimera of prokaryotic ancestors
The chimera of Greek mythology was part goat, part lion, and part serpent.
Similarly, the eukaryotic cell is a chimera of prokaryotic parts:
- mitochondria from one bacteria
- plastids from another
- nuclear genome from the host cell
The search for the closest living prokaryotic relatives to the eukaryotic cell
has been based on molecular comparisons because no morphological homologies connect
species so diverse.
- Sequence comparisons of the small ribosomal subunit RNA (SSU-rRNA) among prokaryotes
and mitochondria have identified the closest relatives of the mitochondria as
the alpha proteobacteria group.
- Sequence comparisons of SSU-rRNA from plastids of eukaryotes and prokaryotes have
indicated a close relationship with cyanobacteria.
While mitochondria and plastids contain DNA and can build proteins, they are not
genetically self-sufficient.
- Some of their proteins are encoded by the organelles' DNA.
- The genes for other proteins are located in the cell's nucleus.
- Other proteins in the organelles are molecular chimeras of polypeptides synthesized
in the organelles and polypeptides imported from the cytoplasm (and ultimately
from nuclear genes).
A reasonable hypothesis for the collaboration between the genomes of the organelles
and the nucleus is that the endosymbionts transferred some of their DNA to the
host genome during the evolutionary transition from symbiosis to integrated eukaryotic
organism.
- Transfer of DNA between modern prokaryotic species is common (for example, by
transformation).
Back to top
4. Secondary endosymbiosis increased the diversity of algae
Taxonomic groups with plastids are scattered throughout the phylogenetic tree
of eukaryotes.
These plastids vary in ultrastructure.
- The chloroplasts of plants and green algae have two membranes.
- The plastids of others have three or four membranes.
- These include the plastids of Euglena (with three membranes) that are
most closely related to heterotrophic species.
The best current explanation for this diversity of plastids is that plastids were
acquired independently several times during the early evolution of eukaryotes.
- Those algal groups with more than two membranes were acquired by secondary
endosymbiosis.
- It was by primary endosymbiosis that certain eukaryotes first acquired
the ancestors of plastids by engulfing cyanobacteria.
- Secondary endosymbiosis occurred when a heterotrophic protist engulfed an algae
containing plastids.
Each endosymbiotic event adds a membrane derived from the vacuole membrane of
the host cell that engulfed the endosymbiont.
In most cases of secondary endosymbiosis, the endosymbiont lost most of its parts,
except its plastid.
In some algae, there are remnants of the secondary endosymbionts.
- For example, the plastids of cryptomonad algae contain vestiges of the endosymbiotic
nucleus, cytoplasm, and even ribosomes.
- Thus, a cryptomonad is a complex chimera, like a box containing a box containing
a box.
Back to top
5. Research on the relationships between the three domains is changing ideas
about the deepest branching in the tree of life
The chimeric origin of the eukaryotic cells contrasts with the classic Darwinian
view of lineal descent through a "vertical" series of ancestors.
- The eukaryotic cell evolved by "horizontal" fusions of species from
different phylogenetic lineages.
- The metaphor of an evolutionary tree starts to break down at the origin of eukaryotes
and other early evolutionary episodes.
The conventional model of relationships among the three domains place the archaea
as more closely related to eukaryotes than they are to prokaryotes.
- Similarities include proteins involved in transcription and translation.
- This model places the host cell in the endosymbiotic origin of eukaryotes as resembling
an early archaean.
The conventional cladogram predicts that the only DNA of bacterial origin in the
nucleus of eukaryotes are genes that were transferred from the endosymbionts that
evolved into mitochondria and plastids.
Surprisingly, systematists have found many DNA sequences in the nuclear genome
of eukaryotes that have no role in mitochondria or chloroplasts.
Also, modern archaea have many genes of bacterial origin.
All three domains seem to have genomes that are chimeric mixes of DNA that was
transferred across the boundaries of the domains.
This has lead some researchers to suggest replacing the classical tree with a
web-like phylogeny
In this new model, the three domains arose from an ancestral community
of primitive cells that swapped DNA promiscuously.
- This explains the chimeric genomes of the three domains.
- Gene transfer across species lines is still common among prokaryotes.
- However, this does not appear to occur in modern eukaryotes.
Back to top
6. The origin of eukaryotes catalyzed a second great wave of diversification
The first great adaptive radiation, the metabolic diversification of the prokaryotes,
set the stage for the second.
The second wave of diversification was catalyzed by the greater structural diversity
of the eukaryotic cell.
The third wave of diversification followed the origin of multicellular bodies
in several eukaryotic lineages.
The diversity of eukaryotes ranges from a great variety of unicellular forms to
such macroscopic, multicellular groups as brown algae, plants, fungi, and animals.
The development of clades among the diverse groups of eukaryotes is based on comparisons
of cell structure, life cycles, and molecules.
- This includes both SSU-rRNA sequences and amino acid sequences for some cytoskeletal
proteins.
If plants, animals, and fungi are designated as kingdoms, then each of the other
major clades of eukaryotes probably deserve kingdom status as well.
- However, protistan systematics is still so unsettled that any kingdom names assigned
to these other clades would be rapidly obsolete.
- In fact, some of the best-known protists, such as the single-celled amoebas, are
not even included in this tentative phylogeny because it is so uncertain where
they fit into the overall eukaryotic tree.
- As tentative as our eukaryotic tree is, the current tree is an effective tool
to organize a survey of the diversity found among protists.
Back to top
C: A Sample of Protistan Diversity
1. Diplomonadida and Parabasala: Diplomonads and parabasalids lack mitochondria
A few protists, including the diplomonds and the parabasalids, lack mitochondria.
According to the "archaezoa hypothesis," these protists are derived
from ancient eukaryotic lineages before the acquisition of endosymbiotic bacteria
that evolved into mitochondria.
- This hypothesis has largely been discarded because of the presence of mitochondrial
genes in the nuclear genomes of both groups.
This evidence suggests a new hypothesis, that these protists lost their
mitochondria during their evolution.
Other details of cell structure and data from molecular systematics still place
the diplomonads and parablastids on the phylogenetic branch that diverged earliest
in eukaryotic history.
The diplomonads have multiple flagella, two separate nuclei, a simply
cytoskeleton, and no mitochondria or plastids.
One example is Giardia lamblia, a parasite that infects the human intestine.
- The most common method of acquiring Giardia is by drinking water contaminated
with feces containing the parasite in a dormant cyst stage.
The parabasalids include trichomonads.
The best known species, Trichomonas vaginalis, inhabits the vagina of
human females.
- It can infect the vaginal lining if the normal acidity of the vagina is disturbed.
- The male urethra may also be infected, but without symptoms.
- Sexual transmission can spread the infection.
Back to top
2. Euglenozoa: The euglenozoa includes both photosynthetic and heterotrophic
flagellates
Several protistan groups, including the euglenoids and kinetoplastids, use flagella
for locomotion.
The euglenoids (Euglenozoa) are characterized by an anterior pocket from which
one or two flagella emerge.
- They also have a unique glucose polymer, paramylon, as a storage molecule.
- While Euglena is chiefly autotrophic, other euglenoids are mixotrophic
or heterotrophic.
The kinetoplastids (Kinoplastida) have a single large mitochondrion
associated with a unique organelle, the kinetoplast.
- The kinetoplast houses extranuclear DNA.
Kinetoplastids are symbiotic and include pathogenic parasites.
For example, Trypanosoma causes African sleeping sickness.
Back to top
3. Alveolata: The alveolata are unicellular protists with subcellular cavities
(alveoli)
The Alveolata combines flagellated protists (dinoflagellates), parasites
(apicomplexans), and ciliated protists (the ciliates).
- This clade has been supported by molecular systematics.
Members of this clade have alveoli, small membrane-bound cavities, under the cell
surface.
- Their function is not known, but they may help stabilize the cell surface and
regulate water and ion content.
The dinoflagellates are abundant components of the phytoplankton that
are suspended near the water surface.
- Dinoflagellates and other phytoplankton form the foundation of most marine and
many freshwater food chains.
- Other species of dinoflagellates are heterotrophic.
- Most dinoflagellates are unicellular, but some are colonial.
Each dinoflagellate species has a characteristic shape, often reinforced by internal
plates of cellulose.
Two flagella sit in perpendicular grooves in the "armor" and produce
a spinning movement.
Dinoflagellate blooms, characterized by explosive population growth, cause red
tides in coastal waters.
- The blooms are brownish-red or pinkish-orange because of the predominant pigments
in the plastids.
- Toxins produced by some red-tide organisms have produced massive invertebrate
and fish kills.
- These toxins can be deadly to humans as well.
One dangerous dinoflagellate, Pfiesteria piscicida, is actually carnivorous.
- This organism produces a toxin that stuns fish.
- The dinoflagellate can then feed on the body fluids of its prey.
- In the past decade, the frequency of Pfiesteria blooms and fish kills
have increased in the mid-Atlantic states of the U.S.
- One hypothesis for this change is an increase in pollution of coastal waters with
fertilizers, especially nitrates and phosphates.
Some dinoflagellates form mutualistic symbioses with cnidarians, animals that
build coral reefs.
- Photosynthetic products from the dinoflagellates provide the main food resource
for reef communities.
Some dinoflagellates are bioluminescent.
- An ATP-driven chemical reaction gives off light when dinoflagellates are disturbed
by water movements.
- The function of bioluminescence may be to attract predators that may eat the smaller
predators that feed on phytoplankton.
All apicomplexans are parasites of animals and some cause serious human
diseases.
- The parasites disseminate as tiny infectious cells (sporozoites)
with a complex of organelles specialized for penetrating host cells
and tissues at the apex of the sporozoite cell.
- Most apicomplexans have intricate life cycles with both sexual and asexual stages
and often require two or more different host species for completion.
Plasmodium, the parasite that causes malaria, spends part of its life
in mosquitoes and part in humans.
The incidence of malaria was greatly diminished in the 1960s by the use of
insecticides against the Anopheles mosquitoes, which spread the disease,
and by drugs that killed the parasites in humans.
- However, resistant varieties of the mosquitoes and the Plasmodium species
have caused a malarial resurgence.
- About 300 million people are infected with malaria in the tropics, and up to 2
million die each year.
Research has had little success in producing a malarial vaccine because Plasmodium
is evasive.
- It spends most of its time inside human liver and blood cells, and continually
changes its surface proteins, continually changing its "face" to the
human immune system.
Identification of a gene that may confer resistance to chloroquine, an antimalarial
drug, may lead to ways to block drug resistance in Plasmodium.
A second promising approach may attack a nonphotosynthetic plastid in Plasmodium.
The Ciliophora (ciliates), a diverse protist group, is named for their
use of cilia to move and feed.
Most ciliates live as solitary cells in freshwater.
Their cilia are associated with a submembrane system of microtubules that may
coordinate movement.
- Some ciliates are completely covered by rows of cilia, whereas others have cilia
clustered into fewer rows or tufts.
- The specific arrangement of cilia adapts the ciliates for their diverse lifestyles.
- Some species have leglike structures constructed from many cilia bonded together,
while others have tightly packed cilia that function as a locomotor membranelle.
In a Paramecium, cilia along the oral groove draw in food that are engulfed
by phagocytosis.
Like other freshwater protists, the hyperosmotic Paramecium expels accumulated
water from the contractile vacuole.
Ciliates have two types of nuclei, a large macronucleus and usually several tiny
micronuclei.
- The macronucleus has 50 or more copies of the genome.
- The macronucleus controls the everyday functions of he cell by synthesizing RNA
and is also necessary for asexual reproduction.
- Ciliated generally reproduce asexually by binary fission of the macronucleus,
rather than mitotic division.
- The micronuclei (with between 1 and 80 copies) are required for sexual processes
that generate genetic variation.
The sexual shuffling of genes occurs during conjugation, during which
micronuclei that have undergone meiosis are exchanged.
- In ciliates, sexual mechanisms of meiosis and syngamy are separate from reproduction.
Back to top
4. Stramenopila: The stramenopila clade includes the water molds and heterokont
algae
The Stramenopila includes both heterotrophic and photosynthetic protists.
- The name of this group is derived from the presence of numerous fine, hairlike
projections on the flagella.
- In most cases a "hairy" flagellum is paired with a smooth flagellum.
- In most stramenopile groups, the only flagellated stage is motile reproductive
cells.
The heterotrophic stramenopiles, the oomycotes, include water molds,
white rusts, and downy mildews.
- Some are unicellular, others have a fine network of coenocytic hyphae (fine, branching
filaments).
- These hyphae have cellulose cells walls and are analogous with the hyphae of true
fungi (with chitin cell walls).
- Unlike fungi, the diploid stage dominates in oomycotes and they have biflagellated
cells.
- These filamentous bodies have extensive surface area, enhancing absorption of
nutrients.
In the Oomycota, the "egg fungi", a relatively large egg cell is fertilized
by a smaller "sperm nucleus," forming a resistant zygote.
Water molds are important decomposers, mainly in fresh water.
- They form cottony masses on dead algae and animals.
- Some water molds are parasitic, growing on the skin and gills of injured fish.
White rusts and downy mildews are parasites of terrestrial plants.
- They are dispersed by windblown spores.
- One species of downy mildew threatened French vineyards in the 1870's and another
species causes late potato blight, which contributed to the Irish famine in the
19th century.
The photosynthetic stramenopile taxa are known collectively as the heterokont
algae.
- "Hetero" refers to the two different types of flagella.
The plastids of these algae evolved by secondary endosymbiosis.
- They have a three-membrane envelope and a small amount of eukaryotic cytoplasm
within the plastid.
- The probable ancestor was a red alga.
The heterokont algae include diatoms, golden algae, and brown algae.
Diatoms (Bacillariophyta) have unique glasslike walls composed of hydrated
silica embedded in an organic matrix.
- The wall is divided into two parts that overlap like a shoe box and lid.
Most of the year, diatoms reproduce asexually by mitosis with each daughter cell
receiving half of the cell wall and regenerating a new second half.
Some species form cysts as resistant stages.
Sexual stages are not common, but sperm may be amoeboid or flagellated, depending
on species.
Diatoms are abundant members of both freshwater and marine plankton.
- Diatoms store food reserves in a glucose polymer, laminarin, and a few store food
as oils.
- Massive accumulations of fossilized diatoms are major constituents of diatomaceous
earth.
Golden algae (Chrysophyta), named for the yellow and brown carotene
and xanthophyll pigments, are typically biflagellated.
Some species are mixotrophic and many live among freshwater and marine plankton.
While most are unicellular, some are colonial.
At high densities, they can form resistant cysts that remain viable for decades.
Brown algae (Phaeophyta) are the largest and most complex algae.
- Most brown algae are multicellular.
- Most species are marine.
Brown algae are especially common along temperate coasts in areas of cool water
and adequate nutrients.
They owe their characteristic brown or olive color to accessory pigments in the
plastids.
Back to top
5. Structural and biochemical adaptations help seaweeds survive and reproduce
at the ocean's margins
The largest marine algae, including brown, red, and green algae, are known
collectively as seaweeds.
Seaweeds inhabit the intertidal and subtidal zones of coastal waters.
- This environment is characterized by extreme physical conditions, including wave
forces and exposure to sun and drying conditions at low tide.
Seaweeds have a complex multicellular anatomy, with some differentiated tissues
and organs that resemble those in plants.
- These analogous features include the thallus or body of the seaweed.
- The thallus typically consists of a rootlike holdfast and a stemlike
stipe, which supports leaflike photosynthetic blades.
Some brown algae have floats to raise the blades toward the surface.
- Giant brown algae, known as kelps, form forests in deeper water.
- The stipes of these plants may be 60 m long.
Many seaweeds have biochemical adaptations for intertidal and subtidal conditions.
- The cells walls, composed of cellulose and gel-forming polysaccharides, help cushion
the thalli against agitation by waves.
Many seaweeds are eaten by coastal people, including Laminaria ("kombu"
in Japan) and Porphyra (Japanese "nori") for sushi wraps.
A variety of gelforming substances are extracted in commercial operations.
- Algin from brown algae and agar and carageenan from red algae are used as thickeners
in food, lubricants in oil drilling, or culture media in microbiology.
Back to top
6. Some algae have life cycles with alternating multicellular haploid and diploid
generations
The multicellular brown, red, and green algae show complex life cycles with
alternation of multicellular haploid and multicellular diploid forms.
- A similar alternation of generations evolved convergently in the life
cycle of plants.
The life cycle of the brown alga Laminaria is an example of alternation
of generations.
The diploid individual, the sporophyte, produces haploid spores (zoospores)
by meiosis.
The haploid individual, the gametophyte, produces gametes by mitosis
that fuse to form a diploid zygote.
In Laminaria, the sporophyte and gametophyte are structurally different,
called heteromorphic.
In other algae, the alternating generations look alike (isomorphic),
but they differ in the number of chromosomes.
Back to top
7. Rhodophyta: Red algae lack flagella
Unlike other eukaryotic algae, red algae have no flagellated stages
in their life cycle.
The red coloration visible in many members is due to the accessory pigment phycoerythrin.
- Coloration varies among species and depends on the depth which they inhabit.
The plastids of red algae evolved from primary endosymbiosis of cyanobacteria.
Some species lack pigmentation and are parasites on other red algae.
Red algae (Rhodophyta) are the most common seaweeds in the warm coastal waters
of tropical oceans.
- Others live in freshwater, still others in soils.
Some red algae inhabit deeper waters than other photosynthetic eukaryotes.
- Their photosynthetic pigments, especially phycobilins, allow some species to absorb
those wavelengths (blues and greens) that penetrate down to deep water.
- One red algal species has been discovered off Bahamas at a depth of over 260m.
Most red algae are multicellular, with some reaching a size to be called "seaweeds".
- The thalli of many species are filamentous.
- The base of the thallus is usually differentiated into a simple holdfast.
The life cycles of red algae are especially diverse.
- In the absence of flagella, fertilization depends entirely on water currents to
bring gametes together.
- Alternation of generation (isomorphic and especially heteromorphic) is common
in red algae.
Back to top
8. Chlorophyta: Green algae and plants evolved from a common photoautotrophic
ancestor
Green algae (chlorophytes and charophyceans) are named for their grass-green
chloroplasts.
- These are similar in ultrastructure and pigment composition to those of plants.
- The common ancestor of green algae and plants probably had chloroplasts derived
from cyanobacteria by primary endosymbiosis.
The charophyceans are especially closely related to land plants.
Most of the 7,000 species of chlorophytes live in freshwater.
- Other species are marine, inhabit damp soil or snow, or live symbiotically within
other eukaryotes.
- Some chlorophytes live symbiotically with fungi to form lichens, a
mutualistic collective.
Chlorophytes range in complexity, including:
- biflagellated unicells that resemble gametes and zoospores
- colonial species and filamentous forms
- multicellular forms large enough to qualify as seaweeds.
Large size and complexity in chlorophytes has evolved by three different mechanisms:
- formation of colonies of individual cells (Volvox)
- the repeated division of nuclei without cytoplasmic division to form multinucleate
filaments (Caulerpa)
- formation of true multicellular forms by cell division and cell differentiation
(Ulva).
Most green algae have both sexual and asexual reproductive stages.
- Most sexual species have biflagellated gametes with cup-shaped chloroplasts.
Photosynthetic protists have evolved in several clades that also have heterotrophic
members.
Different episodes of secondary endosymbiosis account for the diversity of protists
with plastids.
Back to top
9. A diversity of protists use pseudopodia for movement and feeding
Three groups of protists use pseudopodia, cellular extensions, to move
and often to feed.
- Most species are heterotrophs that actively hunt bacteria, other protists, and
detritus.
- Other species are symbiotic, including some human parasites.
- Little is known of their phylogenetic relationships to other protists and they
themselves are distinct eukaryotic lineages.
Rhizopods (amoebas) are all unicellular and use pseudopodia to move
and to feed.
Pseudopodium emerge from anywhere in the cell surface.
- To move, an amoeba extends a pseudopod, anchors its tip, and then streams more
cytoplasm into the pseudopodium.
Amoeboid movement is driven by changes in microtubules and microfilaments in the
cytoskeleton.
Pseudopodia activity is not random but in fact directed toward food.
In some species pseudopodia extend out through openings in a protein shell around
the organism.
Amoebas inhabit freshwater and marine environments
- They may also be abundant in soils.
Most species are free-living heterotrophs.
Some are important parasites.
- These include Entamoeba histolytica which causes amoeboid dysentery
in humans.
- These organisms spread via contaminated drinking water, food, and eating utensils.
Actinopod (heliozoans and radiolarians), "ray foot," refers to slender
pseudopodia (axopodia) that radiate from the body.
- Each axopodium is reinforced by a bundle of microtubules covered by a thin layer
of cytoplasm.
Most actinopods are planktonic.
- The large surface area created by axopodia help them to float and feed.
- Smaller protists and other microorganisms stick to the axopodia and are phagocytized
by the thin layer of cytoplasm.
- Cytoplasmic streaming carries the engulfed prey into the main part of the cell.
Most heliozoans ("sun animals") live in fresh water.
- Their skeletons consist of unfused siliceous (glassy) or chitinous plates.
The term radiolarian refers to several groups of mostly marine actinopods.
- In this group, the siliceous skeleton is fused into one delicate piece.
- After death, these skeleton accumulate as an ooze that may be hundreds of meters
thick in some seafloor locations.
Foraminiferans, or forams, are almost all marine.
- Most live in sand or attach to rocks or algae.
- Some are abundant in the plankton.
- Forams have multichambered, porous shells, consisting of organic materials hardened
with calcium carbonate.
Pseudopodia extend through the pores for swimming, shell formation, and feeding.
- Many forams form symbioses with algae.
Over ninety percent of the described forams are fossils.
- The calcareous skeletons of forams are important components of marine sediments.
- Fossil forams are often used as chronological markers to correlate the ages of
sedimentary rocks from different parts of the world.
Back to top
10. Mycetozoa: Slime molds have structural adaptations and life cycles that
enhance their ecological roles as decomposers
Mycetozoa (slime molds or "fungus animals") are neither fungi
nor animals, but protists.
- Any resemblance to fungi is analogous, not homologous, for their convergent role
in the decomposition of leaf litter and organic debris.
Slime molds feed and move via pseudopodia, like amoeba, but comparisons of protein
sequences place slime molds relatively close to the fungi and animals.
The plasmodial slime molds (Myxogastrida) are brightly pigmented, heterotrophic
organisms.
The feeding stage is an amoeboid mass, the plasmodium, that may be several
centimeters in diameter.
- The plasmodium is not multicellular, but a single mass of cytoplasm with multiple
nuclei.
The diploid nuclei undergo synchronous mitotic divisions, perhaps thousands at
a time.
Within the cytoplasm, cytoplasmic streaming distributes nutrients and oxygen throughout
the plasmodium.
The plasmodium phagocytises food particles from moist soil, leaf mulch, or rotting
logs.
If the habitat begins to dry or if food levels drop, the plasmodium differentiates
into stages that lead to sexual reproduction.
The cellular slime molds (Dictyostelida) straddle the line between individuality
and multicellularity.
- The feeding stage consists of solitary cells.
- When food is scarce, the cells form an aggregate ("slug") that functions
as a unit.
- Each cell retains its identity in the aggregate.
The dominant stage in a cellular slime mold is the haploid stage.
- Aggregates of amoebas form fruiting bodies that produce spores in asexual reproduction.
- Most cellular slime molds lack flagellated stages.
Back to top
11. Multicellularity originated independently many times
The origin of unicellular eukaryotes permitted more structural diversity than
was possible for prokaryotes.
This ignited an explosion of biological diversification.
The evolution of multicellular bodies and the possibility of even greater structural
diversity, triggered another wave of diversification.
Back to top
Course Pages maintained by
Dr. Graeme Lindbeck
.