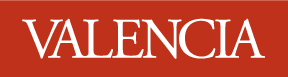
BSC 1010C
General Biology I
Dr.
Graeme Lindbeck
glindbeck@valenciacollege.edu

From Gene to Protein
Outline
- The study of metabolic defects provided evidence that genes specify proteins
- How Genes Control Metabolism
- One Gene-One Polypeptide
- Transcription and translation are the two main steps from gene to protein
- In the genetic code, a particular triplet of nucleotides specifies a certain amino acid
- Cracking the Genetic Code
- The Evolutionary Significance of a Common Genetic Language
- Transcription is the DNA-directed synthesis of RNA
- RNA Polymerase Binding and Initiation of Transcription
- Elongation of the RNA Strand
- Termination of Transcription
- Translation is the RNA-directed synthesis of a polypeptide
- The Structure and Function of Transfer RNA
- Aminoacyl-tRNA Synthetases
- Ribosomes
- Building a Polypeptide
- Polyribosomes
- From Polypeptide to Functional Protein
- Some polypeptides have signal sequences that target them to specific destinations in the cell
- Comparing protein synthesis in prokaryotes and eukaryotes
- Eukaryotic cells modify RNA after transcription
- Alteration of MRNA Ends
- Split Genes and RNA Splicing
- Ribozymes
- The Functional and Evolutionary Importance of Introns
- A point mutation can affect the function of a protein
- Types of Point Mutations
- Mutagens

Inherited instructions in DNA direct protein synthesis. Thus, proteins are
the links between genotype and phenotype, since proteins are directly involved
in the expression of specific phenotypic traits.
Back to top
Archibald Garrod was the first to propose the relationship between genes and proteins (1909).
- He suggested that genes dictate phenotypes through enzymes that catalyze reactions.
- As a physician, Garrod was familiar with inherited diseases which he called "inborn errors in metabolism." He hypothesized that such diseases reflect the patient's inability to make particular enzymes.
- One example he studied was alkaptonuria, which causes the afflicted person's urine to turn black.
Þ People with alkaptonuria accumulate alkapton in their urine, causing it to darken on
contact with air.
Þ Garrod reasoned that alkaptonurics, unlike normal individuals, lack the enzyme that breaks down alkapton.
- How Genes Control Metabolism
Garrod's hypothesis was confirmed several decades later by research which deten-nined that specific genes direct production of specific enzymes.
- Biochemists found that cells synthesize and degrade organic compounds via metabolic pathways, with each sequential step catalyzed by a specific enzyme.
- Geneticists George Beadle and Boris Ephrussi (1930's) studied eye color in Drosophila. They speculated that mutations affecting eye color block pigment synthesis by preventing enzyme production at certain steps in the pigment synthesis pathw
ay.
George Beadle and Edward Tatum were later able to demonstrate the relationship between genes and enzymes by studying mutants of a bread mold, Neurospora crassa.
- Wild-type Neurospora in laboratory colonies can survive on minimal medium. All other molecules needed by the mold are produced by its own metabolic pathways from this minimal nutrient source.
- Beadle and Tatum searched for mutants or auxotrophs that could not survive on minimal medium because they lacked the ability to synthesize essential molecules.
- Mutants were identified by transferring fragments of growing fungi (in complete medium) to vials containing minimal medium. Fragments that didn't grow were identified as auxotrophic mutants.
Auxotroph = (Auxo = to augment; troph = nourishment) Nutritional mutants that can only be grown on minimal medium augmented with nutrients not required by the wild type.
Minimal medium = Support medium that is mixed only with molecules required for the growth of wild-type organisms.
- Minimal medium for Neurospora contains inorganic salts, sucrose and the vitamin, biotin.
- Nutritional mutants cannot survive only on minimal medium.
Complete growth medium = Minimal medium supplemented with all 20 amino acids and some other nutrients.
- Nutritional mutants can grow on complete growth medium, since all essential nutrients are provided.
Beadle and Tatum then identified specific metabolic defects (from mutations) by transferring fragments of auxotrophic mutants growing on complete growth medium to vials containing minimal medium each supplemented with only one additional nutrient.
- Vials where growth occurred indicated the metabolic defect, since the single supplement provided the necessary component.
- For example, if a mutant grew on minimal medium supplemented with only arginine, it could be concluded that the mutant was defective in the arginine synthesis pathway.
Experiment:
Beadle and Tatum experimented further to more specifically describe the defect in the multistep pathway that synthesizes the amino acid arginine.
Arginine synthesis requires three steps each catalyzed by a specific enzyme:
|
Gene A |
|
Gene B |
|
Gene C |
|
|
¯ |
|
¯ |
|
¯ |
|
|
Enzyme A |
|
Enzyme B |
|
Enzyme C |
|
Precursor |
® |
Ornithine |
® |
Citrulline |
® |
Arginine |
They distinguished between three classes of arginine auxotrophs by adding either arginine, citruiline, or ornithine to the medium and seeing if growth occurred.
Results:
Some mutants required arginine, some either arginine or citrulline, and others could grow when any of the three were added.
|
Minimal Medium (MM) |
mm plus Ornithine |
mm plus Citrulline |
mm plus Arginine |
Wild Type | + | + | + | + |
Class I Mutants | - | + | + | + |
Class II Mutants | - | - | + | + |
Class III Mutants | - | - | - | + |
+ = growth, - = no growth
Conclusions:
Beadle and Tatum deduced from their data that the three classes of mutants each lacked a different enzyme and were thus blocked at different steps in the arginine synthesis pathway.
- Class I mutants lacked enzyme A; Class II mutants lacked enzyme B; and Class III mutants lacked enzyme C.
- Assuming that each mutant was defective in a single gene, they formulated the one gene-one enzyme hypothesis, which states that the function of a gene is to dictate the production of a specific enzyme.
- One Gene-One Polypeptide
Beadle and Tatum's one gene-one enzyme hypothesis has been slightly modified:
- While most enzymes are proteins, many proteins are not enzymes. Proteins that are not enzymes are still, nevertheless, gene products.
- Also, many proteins are comprised of two or more polypeptide chains, each chain specified by a different gene (e.g. globulin chains of hemoglobin).
As a result of this new information, Beadle and Tatum's hypothesis has been restated as one gene-one polypeptide.
Back to top
II. Transcription and translation are the two main steps from gene to protein
Ribonucleic acid (RNA) links DNA's genetic instructions for making proteins to the process of protein synthesis. It copies or transcribes the message from DNA and then translates that message into a protein.
- RNA, like DNA, is a nucleic acid or polymer of nucleotides.
- RNA structure differs from DNA in the following ways:
ÞThe five-carbon sugar in RNA nucleotides is ribose rather than deoxyribose.
ÞThe nitrogenous base uracil is found in place of thymine,
The linear sequence of nucleotides in DNA ultimately determines the linear sequence of amino acids in a protein.
- Nucleic acids are made of four types of nucleotides which differ in their nitrogenous bases. Hundreds or thousands of nucleotides long, each gene has a specific linear sequence of the four possible bases.
- Proteins are made of twenty types of amino acids linked in a particular linear sequence (the protein's primary structure).
- Information flows from gene to protein through two major processes, transcription and translation.
Transcription = The synthesis of RNA using DNA as a template.
- A gene's unique nucleotide sequence is transcribed from DNA to a complementary nucleotide sequence in messenger RNA (mRNA).
- The resulting mRNA carries this transcript of protein-building instructions to the cell's protein-synthesizing machinery.
Translation = Synthesis of a polypeptide, which occurs under the direction of messenger RNA (mRNA).
- During this process, the linear sequence of bases in mRNA is translated into the linear sequence of amino acids in a polypeptide.
- Translation occurs on ribosomes, complex particles composed of ribosomal RNA (rRNA)
and protein that facilitate the orderly linking of amino acids into polypeptide chains.
Prokaryotes and eukaryotes differ in how protein synthesis is organized within their cells.
- Prokaryotes lack nuclei, so DNA is not segregated from ribosomes or the protein synthesizing machinery. Thus, transcription and translation occur in rapid succession.
- Eukaryotes have nuclear envelopes that segregate transcription in the nucleus from translation in the cytoplasm; mRNA, the intermediary, is modified before it moves from the nucleus to the cytoplasm where translation occurs. This RNA processing occurs only in eukaryotes.
Back to top
III. In the genetic code, a particular triplet of nucleotides specifies a certain amino acid
There is not a one-to-one correspondence between the nitrogenous bases and the amino acids they specify, since there are only 4 nucleotides and 20 amino acids.
- A two-to-one correspondence of bases to amino acids would only specify 16 (42) of the 20 amino acids.
- A three-to-one correspondence of bases to amino acids would specify 64 (43) amino acids.
Researchers have verified that the flow of information from a gene to a protein is based on a triplet code.
- Triplets of nucleotides are the smallest units of uniform length to allow translation into all 20 amino acids with plenty to spare.
- These three-nucleotide "words" are called codons.
Codon = A three-nucleotide sequence in mRNA that specifies which amino acid will be added to a growing polypeptide or that signals termination; the basic unit of the genetic code.
Genes are not directly translated into amino acids, but are first transcribed as codons into mRNA.
- For each gene, only one of the two DNA strands (the template strand) is transcribed
- The complementary nontemplate strand is the parental strand for making a new template when DNA replicates.
- The same DNA strand can be the template strand for some genes and the nontemplate strand for others.
An mRNA is complementary to the DNA template from which it is transcribed.
- For example, if the triplet nucleotide sequence on the template DNA strand is CCG; GGC, the codon for glycine, will be the complementary mRNA transcript.
During translation, the linear sequence of codons along mRNA is translated into the linear sequence of amino acids in a polypeptide.
- Each mRNA codon specifies which one of 20 amino acids will be incorporated into the corresponding position in a polypeptide.
- Because codons are base triplets, the number of nucleotides making up a genetic message is three times the number of amino acids making up the polypeptide product.
- Cracking the Genetic Code
The first codon was deciphered in 1961 by Marshall Nirenberg of the National Institutes of Health.
- He synthesized an mRNA by linking only uracil-bearing RNA nucleotides, resulting in UUU codons.
- Nirenberg added this "poly U" to a test-tube mixture containing the components necessary for protein synthesis. The artificial mRNA (poly U) was translated into a polypeptide containing a string of only one amino acid, phenylalanine.
- Nirenberg concluded that the mRNA codon UUU specifies the amino acid phenylalanine.
- These same techniques were used to determine amino acids specified by the codons AAA, GGG, and CCC.
More elaborate techniques allowed investigators to determine all 64 codons by the mid1960's.
- 61 of the 64 triplets code for amino acids.
- The triplet AUG has a dual function - it is the start signal for translation and codes for methionine.
- Three codons do not code for amino acids, but signal termination (UAA, UAG and UGA).
There is redundancy in the genetic code, but no ambiguity.
- Redundancy exists since two or more codons differing only in their third base can code for the same amino acid (UUU and UUC both code for phenylalanine).
- Ambiguity is absent, since codons code for only one amino acid.
The correct ordering and grouping of nucleotides is important in the molecular language of cells. This ordering is called the reading frame.
Reading frame = The correct grouping of adjacent nucleotide triplets into codons that are in the correct sequence on mRNA.
- For example, the sequence of amino acids - Trp - Phe - Gly - Arg - Phe - can be assembled in the correct order only if the mRNA codons UGGUUUGGCCGUUUU are read in the correct sequence and groups.
- The cell reads the message in the correct frame as a series of nonoverlapping three-letter words: UGG - UUU - GGC - CGU - ULJU.
- The Evolutionary Significance of a Common Genetic Language
The genetic code is shared nearly universally among living organisms.
- For example, the RNA codon CCG is translated into proline in all organisms whose genetic codes have been examined.
- The technology exists to transfer genes from one species to another. For example, the human gene for insulin can be inserted into bacteria where it is successfully expressed.
There are some exceptions to this universality:
- Several ciliates (e.g. Paramecium and Tetrahymena) depart from standard code; codons UAA and UAG are not stop signals, but code for glutamine.
- Mitochondria and chloroplasts have their own DNA that codes for some proteins.
- Mitochondrial genetic codes vary even among organisms; for example, CUA codes for threonine in yeast mitochondria and leucine in mammalian mitochondria.
The fact that the genetic code is shared nearly universally by all organisms indicates that this code was established very early in life's history.
Back to top
IV. Transcription is the DNA-directed synthesis of RNA
Transcription of messenger RNA (mRNA) from template DNA is catalyzed by RNA polymerases, which:
- Separate the two DNA strands and link RNA nucleotides as they base-pair along the DNA template.
- Add nucteotides only to the 3' end; thus, mRNA molecules grow in the 5'® 3' direction.
There are several types of RNA polymerase.
- Prokaryotes have only one type of RNA polymerase that synthesizes all types of RNA - mRNA, rRNA and tRNA.
- Eukaryotes have three RNA polymerases that transcribe genes. RNA polymerase II is the polymerase that catalyzes mRNA synthesis; it transcribes genes that will be translated into proteins.
Specific DNA nucleotide sequences mark where transcription of a gene begins (initiation) and ends (termination). Initiation and termination sequences plus the nucleotides in between are called a transcription unit.
Transcription unit = Nucleotide sequence on the template strand of DNA that is transcribed into a single RNA molecule by RNA polymerase; it includes the initiation and termination sequences, as well as the nucleotides in between.
- In eukaryotes, a transcription unit contains a single gene, so the resulting mRNA codes for synthesis of only one polypeptide.
- In prokaryotes, a transcription unit can contain several genes, so the resulting mRNA may code for different, but functionally related, proteins.
Transcription occurs in three key steps: a) polymerase binding and initiation; b) elongation; and c) termination.
- RNA Polymerase Binding and Initiation of Transcription
RNA polymerases bind to DNA at regions called promoters.
Promoter = Region of DNA that includes the site where RNA polymerase binds and where transcription begins (initiation site). In eukaryotes, the promoter is about 100 nucleotides long and consists of:
- The initiation site, where transcription begins.
- A few nucleotide sequences recognized by specific DNA-binding proteins (transcription factors) that help initiate transcription.
In eukaryotes, RNA polymerases cannot recognize the promoter without the help of transcription factors.
Transcription factors = DNA-binding proteins that bind to specific DNA nucleotide sequences at the promoter and that help RNA polymerase recognize and bind to the promoter region, so that transcription can begin.
- RNA polymerase II, the enzyme that synthesizes mRNA in eukaryotes, usually cannot recognize a promoter unless a specific transcription factor binds to a region on the promoter called a TATA box.
TATA box = A short nucleotide sequence at the promoter that is rich in thymine (T) and adenine (A) and that is located about 25 nucleotides upstream from the initiation site.
- RNA polymerase II recognizes the complex between the bound TATA transcription factor and the DNA binding site.
- Once RNA polymerase recognizes and attaches to the promoter region, it probably associates with other transcription factors before RNA synthesis begins.
- When active RNA polymerase binds to a promoter, the enzyme separates the two DNA strands at the initiation site, and transcription begins.
- Elongation of the RNA Strand
Once transcription begins, RNA polymerase II moves along DNA and performs two primary functions:
- It untwists and opens a short segment of DNA exposing about ten nucleotide bases; one of the exposed DNA strands is the template for base-pairing with RNA nucleotides.
- It links incoming RNA nucleotides to the 3' end of the elongating strand; thus, RNA grows one nucleotide at a time in the 5' ® 3' direction.
During transcription, mRNA grows about 30 - 60 nucleotides per second. As the mRNA strand elongates:
- It peels away from its DNA template.
- The nontemplate strand of DNA re-forms a DNA - DNA double helix by pairing with the template strand.
Following in series, several molecules of RNA polymerase II can simultaneously transcribe the same gene.
- Cells can thus produce particular proteins in large amounts.
- The growing RNA strands hang free from each polymerase. The length of each strand varies and reflects how far the enzyme has traveled from the initiation site on template DNA.
- Termination of Transcription
Transcription proceeds until RNA polymerase reaches a termination site on the DNA.
Terminator sequence = DNA sequence that signals RNA polymerase to stop transcription and to release the RNA molecule and DNA template.
- Additional proteins may cooperate with RNA polymerase in termination.
- In eukaryotes, the most common terminator sequence is AATAAA.
Prokaryotic mRNA is ready for translation as soon as it leaves the DNA template. Eukaryotic mRNA, however, must be processed before it leaves the nucleus and becomes functional.
Back to top
V. Translation is the RNA-directed synthesis of a polypeptide
During translation, proteins are synthesized according to a genetic message of sequential codons along mRNA.
- Transfer RNA (tRNA) is the interpreter between the two forms of information - base sequence in mRNA and amino acid sequence in polypeptides.
- tRNA aligns the appropriate amino acids to form a new polypeptide. To perform this function, tRNA must:
ÞTransfer amino acids from the cytoplasm's amino acid pool to a ribosome.
ÞRecognize the correct codons in mRNA.
Molecules of tRNA are specific for only one particular amino acid. Each type of tRNA associates a distinct mRNA codon with one of the 20 amino acids used to make proteins.
- One end of a tRNA molecule attaches to a specific amino acid.
- The other end attaches to an mRNA codon by base pairing with its anticodon.
Anticodon = A nucleotide triplet in tRNA that base pairs with a complementary nucleotide triplet (codon) in mRNA.
tRNAs decode the genetic message, codon by codon. For example,
- The mRNA codon UUU is translated as the amino acid phenylalanine.
- The tRNA that transfers phenylalanine to the ribosome has an anticodon of AAA.
- When the codon UUU is presented for translation, phenylalanine will be added to the growing polypeptide.
- As tRNAs deposit amino acids in the correct order, ribosomal enzymes link them into a chain.
- The Structure and Function of Transfer RNA
All types of eukaryotic RNA, including tRNA, are transcribed from template DNA located within the nucleus.
- tRNA must travel from the nucleus to the cytoplasm, where translation occurs.
- Once in the cytoplasm, each tRNA molecule can be used repeatedly.
The ability of tRNA to carry specific amino acids and to recognize the correct codons depends upon its structure; its form fits function.
- tRNA is a single-stranded RNA only about 80 nucleotides long.
- The strand is folded, forming several double-stranded regions where short base sequence hydrogen bond with other complementary base sequences.
- A single-plane view reveals a clover leaf shape.
The three-dimensional structure is roughly L-shaped.
- A loop protrudes at one end of the L and has a specialized sequence of three bases called the anticodon.
- At the other end of the L protrudes the 3' end of the tRNA molecule - the attachment site for an amino acid.
There are only about 45 distinct types of tRNA. However, this is enough to translate the 64 codons, since some tRNAs recognize two or three mRNA codons specifying the same amino acid.
- This is possible because the base-pairing rules are relaxed between the third base of an mRNA codon and the corresponding base of a tRNA anticodon.
- This exception to the base-pairing rule is called wobble.
Wobble = The ability of one tRNA to recognize two or three different mRNA codons; occurs when the third base (5' end) of the tRNA anticodon has some play or wobble, so that it can hydrogen bond with more than one kind of base in the third position
(3' end) of the codon.
- For example, the base U in the wobble position of a tRNA anticodon can pair with either A or G in the third position of an mRNA codon.
- Some tRNAs contain a modified base called inosine (I), which is in the anticodon's wobble position and can base pair with U, C or A in the third position of an mRNA codon.
- Thus, a single tRNA with the anticodon CCI will recognize three mRNA codons: GGU, GGC or GGA - all of which code for glycine.
- Aminoacyl-tRNA Synthetases
The correct linkage between tRNA and its designated amino acid must occur before the anticodon pairs with its complementary mRNA codon. This process of correctly pairing a tRNA with its appropriate amino acid is catalyzed by an aminoacyl-tRNA synthetase.
Aminoacyl-tRNA synthetase = A type of enzyme that catalyzes the attachment of an amino acid to its tRNA.
- Each of the 20 amino acids has a specific aminoacyl-tRNA synthetase.
- an endergonic reaction driven by the hydrolysis of ATP, the proper synthetase attaches an amino acid to its tRNA in two steps:
1. Activation of the amino acid with AMP. The synthetase's active site binds the amino acid and ATP; the ATP loses two phosphate groups and attaches to the amino acid as AMP (adenosine monophosphate).
2. Attachment of the amino acid to tRNA. The appropriate tRNA covalently bonds to the amino acid, displacing AMP from the enzyme's active site.
The aminoacyl-tRNA complex releases from the enzyme and transfers its amino acid to a growing polypeptide on the ribosome.
- Ribosomes
Ribosomes coordinate the pairing of tRNA anticodons to mRNA codons.
- Ribosomes have two subunits (small and large) which are separated when not involved in protein synthesis.
- Ribosomes are composed of about 60% ribosomal RNA (rRNA) and 40% protein.
The large and small subunits of eukaryotic ribosomes are:
- Constructed in the nucleolus.
- Dispatched through nuclear pores to the cytoplasm.
- Once in the cytoplasm, are assembled into functional ribosomes only when attached to an mRNA.
Compared to eukaryotic ribosomes, prokaryotic ribosomes are smaller and have a different molecular composition.
- Selection of effective drug therapies against bacterial pathogens capitalizes on this difference.
- For example, the antibiotics tetracycline and streptomycin can be used to combat bacterial infections, because they inhibit bacterial protein synthesis without afffecting the ribosomes of the eukaryotic host.
In addition to an mRNA binding site, each ribosome has three tRNA binding (P and A).
- The P site holds the tRNA carrying the growing polypeptide chain.
- The A site holds the tRNA carrying the next amino acid to be added.
- Discharged tRNAs exit the ribosome from the E site.
As the ribosome holds the tRNA and mRNA molecules together, enzymes transfer the new amino acid from its tRNA to the carboxyl end of the growing polypeptide.
- Building a Polypeptide
The building of a polypeptide, or translation, occurs in three stages: 1) initiation, 2) elongation, and 3) termination.
- All three stages require enzymes and other protein factors.
- Initiation and elongation also require energy provided by GTP (a molecule closely related to ATP).
1. Initiation
Initiation must bring together the mRNA, the first amino acid attached to its tRNA, and the two ribosomal subunits.
An initiation complex is assembled as mRNA and a special initiator tRNA bind to a small ribosomal subunit.
- In eukaryotes, the small ribosomal subunit binds first to an initiator tRNA with the anti-codon UAC. This tRNA carries methionine, the first amino acid to be added.
- The small ribosomal subunit next binds to the 5' end of mRNA. mRNA has a specific ribosome-recognition sequence at the 5' upstream end that base pairs with a complementary sequence on rRNA.
- With help from the small ribosomal subunit, bound initiator tRNA finds and base pairs with the initiation or start codon on mRNA. This start codon, AUG, marks the place where translation will begin and is located just downstream from the ribosome-recognition sequence.
- Assembly of the initiation complex - small ribosomal subunit, initiator tRNA and mRNA - requires:
ÞProtein initiation factors that are bound to the small ribosomal subunit.
ÞOne GTP molecule that probably stabilizes the binding of initiation factors and upon hydrolysis drives the attachment of the large ribosomal subunit.
In the second step, a large ribosomal subunit binds to the small one to form a functional ribosome.
- Initiation factors attached to the small ribosomal subunit are released, allowing the large subunit to bind with the small subunit.
- The initiator tRNA fits into the P site on the ribosome.
- The vacant A site is ready for the next aminoacyl-tRNA.
2. Elongation
Several proteins called elongation factors take part in this three-step cycle which adds amino acids one by one to the initial amino acid.
- Codon recognition. The mRNA codon in the A site of the ribosome forms hydrogen bonds with the anticodon of an entering tRNA carrying the next amino acid in the chain.
- An elongation factor directs tRNA into the A site.
- Hydrolysis of GTP provides energy for this step.
- Peptide bond formation. An enzyme, peptidyl transferase, catalyzes the formation of a peptide bond between the polypeptide in the P site and the new amino acid in the A site.
- Peptidyl transferase is part of the large ribosomal subunit and consists of ribosomal proteins and rRNA.
- The polypeptide separates from its tRNA and is transferred to the new amino acid carried by the tRNA in the A site.
- Translocation. The tRNA in the A site, which is now attached to the growing polypeptide, is translocated to the P site. Simultaneously, the tRNA that was in the P site is translocated to the E site and from there exits the ribosome.
- During this process, the codon and anticodon remain bonded, so the mRNA and the tRNA move as a unit, bringing the next codon to be translated into the A site.
- The mRNA is moved through the ribosome only in the 5' to 3' direction. GTP hydrolysis provides energy for each translocation step.
- Termination
Each iteration of the elongation cycle takes about 60 milliseconds and is repeated until synthesis is complete and a termination codon reaches the ribosome's A site.
Termination codon (stop codon) = Base triplet (codon) on mRNA that signals the end of translation.
- Stop codons are UAA, UAG and UGA.
- Stop codons do not code for amino acids.
When a stop codon reaches the ribosome's A site, a protein release factor binds to the codon and initiates the following sequence of events:
- Peptidyl transferase hydrolyzes the bond between the completed polypeptide and the tRNA in the P site.
- This frees the polypeptide and tRNA, so they can both release from the ribosome.
- The two ribosomal subunits dissociate from mRNA and separate back into a small and a large subunit.
- Polyribosomes
Single ribosomes can make average-sized polypeptides in less than a minute; usually, however, clusters of ribosomes simultaneously translate an mRNA.
Polyribosome = A cluster of ribosomes simultaneously translating an mRNA molecule.
- Once a ribosome passes the initiation codon, a second ribosome can attach to the mRNA.
- Several ribosomes may translate an mRNA at once, making many copies of a polypeptide.
- From Polypeptide to Functional Protein
The biological activity of proteins depends upon a precise folding of the polypeptide chain into a native three-dimensional conformation.
- Genes determine primary structure, the linear sequence of amino acids.
- Primary structure determines how a polypeptide chain will spontaneously coil and fold to form a three-dimensional molecule with secondary and tertiary structure.
Some proteins must undergo post-translational modification before they become fully functional in the cell.
- Sugars, lipids, phosphate groups, or other additives may be attached to some amino acids.
- One or more amino acids may be enzymatically cleaved from the leading (amino) end of the polypeptide chain.
- Single polypeptide chains may be divided into two or more pieces (e.g. insulin).
- Two or more polypeptides may join as subunits of a protein that has quaternary structure.
Back to top
VI. Some polypeptides have signal sequences that target them to specific destinations in the cell
Eukaryotic ribosomes function either free in the cytosol or bound to endomembranes.
- Bound and free ribosomes are structurally identical and interchangeable.
- Most proteins made by free ribosomes will function in the cytosol.
- Attached to the outside of the endoplasmic reticulum, bound ribosomes generally make proteins that are:
Þdestined for membrane inclusion in endomembrane system (e.g. nuclear envelope, ER, Golgi, lysosomes, vacuoles and plasma membrane).
Þsecretary proteins destined for export.
There is only one type of ribosome, and synthesis of all proteins begins in the cytosol. What determines whether a ribosome will be free in the cytosol or attached to rough ER?
- Messenger RNA for secretary proteins codes for an initial signal sequence of 16 - 20 hydrophobic amino acids at the amino end of the forming polypeptide.
- When a ribosome begins to synthesize a protein with a signal sequence, it moves to the ER membrane by a mechanism that involves two other components.
ÞSignal recognition particle (SRP). This protein complex moves between the ER membrane and the cytosol. It is an adaptor that attaches both to the signal sequence of a growing polypeptide and to a receptor protein in the ER membrane (SRP receptor).
ÞSRP receptor. This receptor protein is built into the ER membrane. The signal recognition particle docks with the receptor, and the ribosome thus becomes bound to the ER membrane.
The ribosome continues protein synthesis and the leading end of the new polypeptide (N-terminus) threads into the cisternal space.
- The signal sequence is removed by an enzyme.
- Newly formed polypeptide is released from the ribosome and folds into its native conformation.
- If an mRNA does not code for a signal sequence, the ribosome remains free and synthesizes its protein in the cytosol.
Different signal sequences may also dispatch proteins to specific sites other than the ER. For example, newly formed proteins may be targeted for mitochondria or chloroplasts.
Back to top
VII. Comparing protein synthesis in prokaryotes and eukaryotes
Transcription and translation are similar in prokaryotes and eukaryotes, but protein synthesis is organized differently within their cells.
- Prokaryotes lack nuclei, so transcription is not segregated from translation; consequently, translation may begin as soon as the 5' end of mRNA peels away from template DNA, even before transcription is complete.
- The significance of a eukaryotic cell's compartmental organization is that transcription and translation are segregated by the nuclear envelope. This allows mRNA to be modified before it moves from the nucleus to the cytoplasm. Such RNA processing occurs only in eukaryotes.
Back to top
VIII. Eukaryotic cells modify RNA after transcription
Before eukaryotic mRNA is exported from the nucleus, it is processed in two ways: a) both ends are covalently altered and b) intervening sequences are removed and the remainder spliced together.
- Alteration of mRNA
During mRNA processing, both the 5' and 3' ends are covalently modified.
5' cap = Modified guanine nucleotide (guanosine triphosphate) that is added to the 5' end of mRNA shortly after transcription begins; has two important functions:
- Protects the growing mRNA from degradation by hydrolytic enzymes.
- Helps small ribosomal subunits recognize the attachment site on mRNA's 5' end. A leader segment of mRNA may also be part of the ribosome recognition signal.
Leader sequence = Noncoding (untranslated) sequence of mRNA from the 5' end to the start codon.
The 3' end, which is transcribed last, is modified by enzymatic addition of a poly-A tail, before the mRNA exits the nucleus.
Poly-A tail = Sequence of about 200 adenine nucleotides added to the 3' end of mRNA before it exits the nucleus.
- May inhibit degradation of mRNA in the cytoplasm.
- May regulate protein synthesis by facilitating mRNA's export from the nucleus to the cytoplasm.
- Is not attached directly to the stop codon, but to an untranslated trailer segment of mRNA.
Trailer sequence = Noncoding (untranslated) sequence of mRNA from the stop codon to the poly-A tail.
- Split Genes and RNA Splicing
The original RNA transcript accurately reflects the complementary base sequence of the gene in template DNA; however, it is much longer than the mRNA that functions in the cytoplasm.
- The original transcript, or precursor mRNA, is heterogeneous nuclear RNA.
heteogeous nuclear RNA (hnRNA) = Pool of RNA in the nucleus that contains molecules of widely varied sizes; includes primary mRNA transcripts or precursor mRNA (pre-mRNA).
- After RNA processing, only a small portion of the original transcript (pre-mRNA) leaves the nucleus as mRNA.
Genes that code for proteins in eukaryotes are not continuous sequences.
- Coding sequences of a gene are interrupted by noncoding segments of DNA called intervening sequences, or introns.
Introns = Noncoding sequences in DNA that intervene between coding sequences (exons); are initially transcribed, but not translated, because they are excised from the transcript before mature RNA leaves the nucleus.
- Coding sequences of a gene are called exons, because they are eventually expressed (translated into protein).
Exons = Coding sequences of a gene that are transcribed and expressed.
- In 1977, Richard Roberts and Philip Sharp independently found evidence for "split genes"; they won the 1993 Nobel Prize for their discovery.
Introns and exons are both transcribed to form hnRNA, but the introns are subsequently removed and the remaining exons linked together during the process of RNA splicing.
RNA splicing = RNA processing that removes introns and joins exons from eukaryotic hnRNA; produces mature mRNA that will move into the cytoplasm from the nucleus.
- Enzymes excise introns and splice together exons to form an mRNA with a continuous coding sequence.
- RNA splicing also occurs during post-transcriptional processing of tRNA and rRNA.
Though there is much left to be discovered, some details of RNA splicing are now known.
- Each end of an intron has short boundary sequences that accurately signal the RNA splicing sites.
- Small nuclear ribonucleoproteins (snRNPs), play a key role in RNA splicing.
Small nuclear ribonucleoproteins (snRNPs) = Complexes of proteins and small nuclear RNAs that are found only in the nucleus; some participate in RNA splicing. (SnRNPs is pronounced "snurps".)
These small nuclear particles are composed of-.
- Small nuclear RNA (snRNA). This small RNA molecule has less than 300 nucleotides - much shorter than mRNA.
- Protein. Each snRNP has seven or more proteins.
- There are various types of snRNPs with different functions; those involved in RNA splicing are part of a larger, more complex assembly called a spliceosome.
Spliceosome = A large molecular complex that catalyzes RNA splicing reactions; composed of small nuclear ribonucleoproteins (snRNPs) and other proteins.
- As the spliceosome is assembled, one type of snRNP base pairs with a complementary sequence at the 5' end of the intron.
- The spliceosome precisely cuts the RNA transcript at specific splice sites at either end of the intron, which is excised as a lariat-shaped loop.
- The intron is released and the adjacent exons are immediately spliced together by the spliceosome.
- Ribozymes
Other kinds of RNA transcripts, such as tRNA and rRNA, are spliced differently; however, as with mRNA splicing, RNA is often involved in catalyzing the reactions.
Ribozymes = RNA molecules that can catalyze reactions by breaking and forming covalent bonds; are called ribozymes to emphasize their enzymelike catalytic activity.
- Ribozymes were first discovered in Tetrahymena, a ciliated protozoan that has selfsplicing rRNA. That is, intron rRNA catalyzes its own splicing, completely without proteins or extra RNA molecules.
- Since RNA is acting as an enzyme, it can no longer be said that "All enzymes are proteins".
- It has since been discovered that rRNA also functions as an enzyme during translation.
Three-dimensional conformations vary among the types of RNA. These differences in shape give RNA its ability to perform a variety of functions, such as:
- Information carrier. Messenger RNA (mRNA) carries genetic information from DNA to ribosomes; this genetic message specifies a protein's primary structure.
- Adaptor molecule. Transfer RNA (tRNA) acts as an adaptor in protein synthesis by translating information from one form (mRNA nucleotide sequence) into another (protein amino acid sequence).
- Catalyst and structural molecule. During translation, ribosomal RNA (rRNA) plays structural and probably enzymatic roles in ribosomes. Small nuclear RNA (snRNA) in snRNP particles also plays structural and enzymatic roles within spliceosomes that
catalyze RNA splicing reactions.
- Viral genomes. Some viruses use RNA as their genetic material.
- The Functional and Evolutionary Importance of Introns
What are the iologicalfunctions of introns and gene splicing?
Introns may play a regulatory role in the cell.
- Intron DNA sequences may control gene activity.
- The splicing process itself may regulate the export of mRNA to the cytoplasm.
Introns may allow a single gene to direct the synthesis of different proteins.
- This can occur if the same RNA transcript is processed differently among various cell types in the same organism.
- For example, all introns may be removed from a particular transcript in one case; but in another, one or more of the introns may be left in place to be translated. Thus, the resulting proteins in each case would be different.
Introns play an important role in the evolution of protein diversity; they increase the probability that recombination of exons will occur between alleles.
- In split genes, coding sequences can be separated by long distances, so they have higher recombination frequencies than continuously coded genes without introns.
- Exons of a "split gene" may code for different domains of a protein that have specific functions, such as, an enzyme's active site or a protein's binding site.
Protein domains = Continuous polypeptide sequences that are structural and functional units in proteins with a modular architecture.
- Genetic recombination can occur in just one exon resulting in the synthesis of a novel protein with only one altered domain.
Back to top
IX. A point mutation can affect the function of a protein
Knowing how genes are translated into proteins, scientists can give a molecular description of heritable changes that occur in organisms.
Mutation = A permanent change in DNA that can involve large chromosomal regions or a single nucleotide pair.
Point mutation = A mutation limited to about one or two nucleotides in a single gene.
- Types of Point Mutations
There are two categories of point mutations: 1) base-pair substitutions and 2) base-pair insertions or deletions.
- Substitutions
Base-pair substitution = The replacement of one base pair with another; occurs when a nucleotide and its partner from the complementary DNA strand are replaced with another pair of nucleotides according to base-pairing rules.
Depending on how base-pair substitutions are translated, they can result in little or no change in the protein encoded by the mutated gene.
- Redundancy in the genetic code is why some substitution mutations have no effect. A base pair change may simply transform one codon into another that codes for the same amino acid.
- Even if the substitution alters an amino acid, the new amino acid may have similar properties to the one it replaces, or it may be in a part of the protein where the exact amino acid sequence is not essential to its activity.
Some base-pair substitutions result in readily detectable changes in proteins.
- Alteration of a single amino acid in a crucial area of a protein will significantly alter protein activity.
- On rare occasions, such a mutation will produce a protein that is improved or has capabilities that enhance success of the mutant organism and its descendants.
- More often, such mutations produce a less active or inactive protein that impairs cell function.
Base-pair substitutions are usually missense mutations or nonsense mutations.
Missense mutation = Base-pair substitution that alters an amino acid codon (sense codon) to a new codon that codes for a different amino acid.
- Altered codons make sense (are translated), but not necessarily that originally intended.
- Base-pair substitutions are usually missense mutations.
Nonsense mutation = Base-pair substitution that changes an amino acid codon (sense codon) to a chain termination codon, or vice versa.
- Nonsense mutations can result in premature termination of translation and the production of a shorter than normal polypeptide.
- Nearly all nonsense mutations lead to nonfunctional proteins.
- Insertions or Deletions
Base-pair insertions or deletions usually have a greater negative effect on proteins than substitutions.
Base-pair insertion = The insertion of one or more nucleotide pairs into a gene.
Base-pair deletion = The deletion of one or more nucleotide pairs from a gene.
Because mRNA is read as a series of triplets during translation, insertion or deletion of nucleotides may alter the reading frame (triplet grouping) of the genetic message. This type of frameshift mutation will occur whenever the number of nucle
otides inserted or deleted is not 3 or a multiple of 3.
Frameshift mutation = A base-pair insertion or deletion that causes a shift in the reading frame, so that codons beyond the mutation will be the wrong grouping of triplets and will specify the wrong amino acids.
- A frameshift mutation causes the nucleotides following the insertion or deletion to be improperly grouped into codons.
- This results in extensive missense, which will sooner or later end in nonsense (premature termination).
- Frameshift will produce a nonfunctional protein unless the insertion or deletion is very near the end of the gene.
- Mutagens
Mutagenesis = The creation of mutations.
- Mutations can occur as errors in DNA replication, repair or recombination that result in base-pair substitutions, insertions or deletions.
- Mutagenesis may be a naturally occurring event causing spontaneous mutations, or mutations may be caused by exposure to mutagens.
Mutagen = Physical or chemical agents that interact with DNA to cause mutations.
- Radiation is the most common physical mutagen in nature and has been used in the laboratory to induce mutations.
- Several categories of chemical mutagens are known including base analogues, which are chemicals that mimic normal DNA bases, but base pair incorrectly.
- The Ames test, developed by Bruce Ames, is one of the most widely used tests for measuring the mutagenic strength of various chemicals. Since most mutagens are carcinogenic, this test is also used to screen for chemical carcinogens.
Course Pages maintained by
Dr. Graeme Lindbeck
.