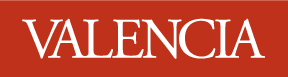
BSC 1010C
General Biology I
Dr.
Graeme Lindbeck
glindbeck@valenciacollege.edu

The Molecular Basis of Inheritance
Outline
- The Search for the genetic material led to DNA
- Evidence That DNA Can Transform Bacteria
- Evidence That Viral DNA Can Program Cells
- Additional Evidence That DNA Is the Genetic Material of Cells
- Watson and Crick discovered the double helix by building models to conform to X-ray data
- During DNA replication, base-pairing enables existing DNA strands to serve as templates for new complementary strands
- A team of enzymes and other proteins functions in DNA replication
- Getting Started: Origins of Replication
- Elongating a New DNA Strand
- Enzymes proofread DNA during its-replication and repair damage to existing DNA
Deoxyribonucleic acid or DNA is the genetic material - Mendel's heritable factors and Morgan's genes on chromosomes. Inheritance has its molecular basis in the precise replication and transmission of DNA from parent to offspring.
Back to top
I. The search for the genetic material led to DNA
By the 1940's, scientists knew that chromosomes carry hereditary material and consist of DNA and protein. Most researchers thought protein was the genetic material because:
- Proteins are macromolecules with great heterogeneity and functional specificity.
- Little was known about nucleic acids.
- The physical and chemical properties of DNA seemed too uniform to account for the multitude of inherited traits.
- Evidence That DNA Can Transform Bacteria
In 1928, Frederick Griffith performed experiments which provided evidence that genetic material is a specific molecule
Griffith was trying to find a vaccine against Streptococcus pneumoniae, a bacterium that causes pneumonia in mammals. He knew that:
- There are two distinguishable strains of the pneumococcus: one produces smooth colonies (S) and the other rough colonies (R).
- Cells of the smooth strain are encapsulated with a polysaccharide coat and cells of the rough strain are not.
- These alternative phenotypes (S and R) are inherited.
Griffith performed four sets of experiments:
Experiment: Griffith injected live S strain of Streptococcus pneumoniae into mice.
- Results: Mice died of pneumonia.
- Conclusions: Encapsulated strain is pathogenic.
Experiment: Mice were injected with live R strain.
- Results: Mice survived and were healthy.
- Conclusions: The bacterial strain lacking the polysaccharide coat was nonpathogenic.
Experiment: Mice were injected with heat-killed S strain of pneumococcus.
- Results: Mice survived and were healthy.
- Conclusions: Polysaccharide coat does not cause pneumonia because it is still present in heat-killed bacteria which proved to be non-pathogenic.
Experiment: Heat-killed S cells mixed with live R cells were injected into mice.
- Results: Mice developed pneumonia and died. Blood samples from dead mice contained live S cells.
- Conclusions: R cells had acquired from the dead S cells the ability to make polysaccharide coats. Griffith cultured S cells from the dead mice. Since the dividing bacteria produced encapsulated daughter cells, he concluded that this newly acqui
red trait was inheritable. This phenomenon is now called transformation.
Transformation = The assimilation of external genetic material by a cell.
What was the chemical nature of the transforming agent?
- Griffith was unable to answer this question, but other scientists continued the search.
- Griffith's experiments hinted that protein is not the genetic material. Heat denatures protein, yet it did not destroy the transforming ability of the genetic material in the heat-killed S cells.
- In 1944, after a decade of research, Oswald Avery, Maclyn McCarty and Colin MacLeod discovered that the transforming agent had to be DNA.
- The discovery by Avery and his coworkers was met with skepticism by other scientists, because they still believed protein was a better candidate for the genetic material and so little was known about DNA.
- Evidence That Viral DNA Can Program Cells
More evidence that DNA is the genetic material came from studies of bacteriophages.
Bacteriophage (phage) = Virus that infects bacteria.
In 1952, Alfred Hershey and Martha Chase discovered that DNA is the genetic material of a phage known as T2. They knew that T2:
- Is one of many phages to infect the enteric bacterium Escherichia coli (E. coli).
- Like other viruses, is little more than DNA enclosed by a protein coat.
- Can quickly reprogram an E. coli cell to produce T2 phages and release the viruses when the cell lyses.
What Hershey and Chase did not know is which viral component - DNA or protein - was responsible for reprogramming the host bacterial cell. They answered this question by performing the following experiment:
Experiment:
- Step 1: Viral protein and DNA were tagged with different radioactive isotopes.
Þ Protein Tagging: T2 and E. coli were grown in media with radioactive sulfur ( 35S) which incorporated only into the phage protein.
Þ DNA Tagging: T2 and E. coli were grown in media containing radioactive phosphorus (32P) which was incorporated only into the phage DNA.
- Step 2: Protein-labeled and DNA-labeled T2 phages were allowed to infect separate samples of nonradioactive E. coli cells.
- Step 3: Cultures were agitated to shake loose phages that remained outside the bacterial cells.
- Step 4: Mixtures were centrifuged forcing the heavier bacterial cells into a pellet on the bottom of the tubes. The lighter viruses remained in the supernatant.
- Step 5: Radioactivity in the pellet and supernatant was measured and compared.
Results:
- In tubes with E coli infected with protein-labeled T2, most of the radioactivity was in the supernatant with viruses.
- In tubes with E coli infected with DNA-labeled T2, most of the radioactivity was in the pellet with the bacterial cells.
- When the bacteria containing DNA-labeled phages were returned to culture medium, the bacteria released phage progeny which contained 32P in their DNA.
Conclusions:
- Viral proteins remain outside the host cell.
- Viral DNA is injected into the host cell.
- Injected DNA molecules cause cells to produce additional viruses with more viral DNA and proteins.
- These data provided evidence that nucleic acids rather than proteins are the hereditary material.
- Additional Evidence That DNA Is the Genetic Material of Cells
Hershey and Chase's experiments provided evidence that DNA is the hereditary material in viruses. Additional evidence pointed to DNA as the genetic material in eukaryotes as well.
Some circumstantial evidence was:
- A eukaryotic cell doubles its DNA content prior to mitosis.
- During mitosis, the doubled DNA is equally divided between two daughter cells.
- An organism's diploid cells have twice the DNA as its haploid gametes.
Experimental evidence for DNA as the hereditary material in eukaryotes came from the laboratory of Erwin Chargaff. In 1947, he analyzed the DNA composition of different organisms.
Using paper chromatography to separate nitrogenous bases, Chargaff reported the following:
- DNA composition is species-specific.
Þ The amount and ratios of nitrogenous bases vary from one species to another.
Þ This source of molecular diversity made it more credible that DNA is the genetic material.
- In every species he studied, there was a regularity in base ratios.
Þ The number of adenine (A) residues approximately equaled the number of thymines (T), and the number of guanines (G) equaled the number of cytosines (C).
Þ The A=T and G=C equalities became known later as Chargaff's rules. The explanation for these rules came with Watson and Crick's structural model for DNA.
Back to top
II. Watson and Crick discovered the double helix by building models to conform to Xray data
By the 1950's, DNA was accepted as the genetic material, and the covalent arrangement in a nucleic acid polymer was well established. The three dimensional structure of DNA, however, was yet to be discovered. Among scientists working on the problem were:
- Linus Pauling - California Institute of Technology
- Maurice Wilkins and Rosalind Franklin - King's College in London
- James D. Watson (American) and Francis Crick - Cambridge University
James Watson went to Cambridge to work with Francis Crick who was studying protein structure with X-ray crystallography.
Watson saw an X-ray photo of DNA produced by Rosalind Franklin at King's College, London. Watson and Crick deduced from Franklin's X-ray data that:
- DNA is a helix with a uniform width of 2 nm. This width suggested that it had two strands.
- Purine and pyrimidine bases are stacked 0.34 nm apart.
- The helix makes one full turn every 3.4 nm along its length.
- There are ten layers of nitrogenous base pairs in each turn of the helix.
Watson and Crick built scale models of a double helix that would conform to the X-ray data and the known chemistry of DNA.
- One of their unsuccessful attempts placed the sugar-phosphate chains inside the molecule.
- Watson next put the sugar-phosphate chains on the outside which allowed the more hydrophobic nitrogenous bases to swivel to the interior away from the aqueous medium.
- Their proposed structure is a ladder-like molecule twisted into a spiral, with sugar-phosphate backbones as uprights and pairs of nitrogenous bases as rungs.
- The two sugar-phosphate backbones of the helix are antiparallel; that is, they run in opposite directions.
Watson and Crick finally solved the problem of DNA structure by proposing that there is a specific pairing between nitrogenous bases. After considering several arrangements, they concluded:
- To be consistent with a 2 nm width, a purine on one strand must pair (by hydrogen bonding) with a pyrimidine on the other.
- Base structure dictates which, pairs of bases can hydrogen bond. The base pairing rule is that adenine can only pair with thymine, and guanine with cytosine.
Purines |
Pyrimidines |
Possible
Base Pairs |
Number of
Hydrogen Bonds |
Adenine (A) |
Thymine (T) |
A-T |
2 |
Guanine (G) |
Cytosine (C) |
G-C |
3 |
The base-pairing rule is significant because:
- It explains Chargaff s rules. Since A must pair with T, their amounts in a given DNA molecule will be about the same. Similarly, the amount of G equals the amount of C.
- It suggests the general mechanisms for DNA replication. If bases form specific pairs, the information on one strand complements that along the other.
- It dictates the combination of complementary base pairs, but places no restriction on the linear sequence of nucleotides along the length of a DNA strand. The sequence of bases can be highly variable which makes it suitable for coding geneti
c information.
- Though hydrogen bonds between paired bases are weak bonds, collectively they stabilize the DNA molecule. Van der Waals forces between stacked bases also help stabilize DNA.
Back to top
III. During DNA replication, base-pairing enables existing DNA strands to serve as templates for new complementary strands
In April 1953, Watson and Crick's new model for DNA structure, the double helix, was published in the British journal Nature. This model of DNA structure suggested a template mechanism for DNA replication.
- Watson and Crick proposed that genes on the original DNA strand are copied by a specific pairing of complementary bases, which creates a complementary DNA strand.
- The complementary strand can then function as a template to produce a copy of the original strand.
In a second paper, Watson and Crick proposed that during DNA replication:
- The two DNA strands separate.
- Each strand is a template for assembling a complementary strand.
- Nucleotides line up singly along the template strand in accordance with the base-pairing rules (A-T and G-C).
- Enzymes link the nucleotides together at their sugar-phosphate groups.
Watson and Crick's model is a semiconservative model for DNA replication.
- They predicted that when a double helix replicates, each of the two daughter molecules will have one old or conserved strand from the parent molecule and one newly created strand.
- In the late 1950's, Matthew Meselson and Franklin Stahl provided the experimental evidence to support the semiconservative model of DNA replication. A brief description of the experimental steps follows.
Hypotheses:
There were three alternate hypotheses for the pattern of DNA replication:
- If DNA replication is conservative, then the parental double helix should remain intact and the second DNA molecule should be constructed as entirely new DNA.
- If DNA replication is semiconservative, then each of the two resulting DNA molecules should be composed of one original or conserved strand (template) and one newly created strand.
- If DNA replication is dispersive, then both strands of the two newly produced DNA molecules should contain a mixture of old and new DNA.
Experiment:
- Step 1: Labeling DNA strands with 15N.
E coli was grown for several generations in a medium with heavy nitrogen ( 15N). As E. coli cells reproduced, they incorporated the 15N into their nitrogen
ous bases.
- Step 2: Transfer of E. coli to a medium with 14N.
E coli cells grown in heavy medium were transferred to a medium with light nitrogen, 14N. There were three-experimental classes of DNA based upon times after
the shift from heavy to light medium:
- Parental DNA from E. coli cells grown in heavy medium.
- First-generation DNA extracted from E. coli after one generation of growth in the light medium.
- Second-generation DNA extracted from E. coli after two replications in the light medium.
- Step 3: Separation of DNA classes based upon density differences.
Meselson and Stahl used a new technique to separate DNA based on density differences between 14N and 15N.
Isolated DNA was mixed with a CsCl solution and placed in an ultracentrifuge. |
¯ |
DNA-CsCl solution was centrifuged at high speed for several days. |
¯ |
Centrifugal force created a CsCl gradient with increased concentration toward the bottom of the centrifuge tube. |
¯ |
DNA molecules moved to a position in the tube where their density equaled that of theCsCl solution. Heavier DNA molecules were closer to the bottom and lighter DNA molecules were closer to the top where the CsCl solution was less den
se.
|
Results:
- DNA from E. coli grown with 15N was heavier than DNA containing the more common, lighter isotope, 14N.
- First-generation DNA after one generation of bacterial growth, was all of intermediate density.
- Second generation DNA after two generations of bacterial growth in light medium was of intermediate and light density.
Conclusions:
- Their results supported the hypothesis of semiconservative replication for DNA.
- The first generation DNA was all hybrid DNA containing one heavy parental strand and one newly synthesized light strand.
- These results were predicted by the semiconservative model. If DNA replication were conservative, two classes of DNA would be produced. The heavy parental DNA would be conserved and newly synthesized DNA would be light. There would be no i
ntermediate density hybrid DNA.
- The results for first-generation DNA eliminated the possibility of conservative replication, but did not eliminate the possibility of dispersive replication.
- Consequently, Meselson and Stahl examined second-generation DNA. The fact that there were two bands, one light and one hybrid band supported the semiconservative model and allowed the investigators to rule out dispersive replication. If DNA
replication were dispersive, there would have been only one band intermediate in density between light and hybrid DNA.
Back to top
IV. A team of enzymes and other proteins functions in DNA replication
The general mechanism of DNA replication is conceptually simple, but the actual process:
- Is complex. The helical molecule must untwist while it copies its two antiparaliel strands simultaneously. This requires the cooperation of over a dozen enzymes and other proteins.
- Is extremely rapid. In prokaryotes, up to 500 nucleotides are added per second. It takes only a few hours to copy the 6 billion bases of a single human cell.
- Is accurate. Only about one in a billion nucleotides is incorrectly paired.
- Getting Started: Origins of Replication
DNA replication begins at special sites called origins of replication that have a specific sequence of nucleotides.
- Specific proteins required to initiate replication bind to each origin.
- The DNA double helix opens at the origin and replication forks spread in both directions away from the central initiation point creating a replication bubble.
- Bacterial or viral DNA molecules have only one replication origin.
- Eukaryotic chromosomes have hundreds or thousands of replication origins. The many replication bubbles formed by this process, eventually merge forming two continuous DNA molecules.
Replication forks = The Y-shaped regions of replicating DNA molecules where new strands are growing.
- Elongating a New DNA Strand
- Strand Separation. There are two types of proteins involved in the separation of parental DNA strands:
- Helicases are enzymes which catalyze unwinding of the parental double helix to expose the template.
- Single-strand binding proteins are proteins which keep the separated strands apart and stabilize the unwound DNA until new complementary strands can be synthesized.
- Synthesis of the New DNA Strands Enzymes called DNA polymerases catalyze synthesis of a new DNA strand.
- According to base-pairing rules, new nucleotides align themselves along the templates of the old DNA strands.
- DNA polymerase links the nucleotides to the growing strand. These strands grow in the 5' ® 3' direction since new nucleotides are added only to the 3' end of the growing strand.
Hydrolysis of nucleoside triphosphates provides the energy necessary to synthesize the new DNA strands.
Nucleoside triphosphate = Nucleotides with a triphosphate (three phosphates) covalently linked to the 5' carbon of the pentose.
- Recall that the pentose in DNA is deoxyribose, and the pentose in RNA is ribose.
- Nucleoside triphosphates that are the building blocks for DNA, lose two phosphates (pyrophosphate group) when they form covalent linkages to the growing chain.
- Exergonic hydrolysis of this phosphate bond drives the endergonic synthesis of DNA; it provides the required energy to form the new covalent linkages between nucleotides.
Continuous synthesis of both DNA strands at a replication fork is not possible, because:
- The sugar phosphate backbones of the two complementary DNA strands run in opposite directions; that is, they are antiparallel.
- Recall that each DNA strand has a distinct polarity. At one end (3' end), a hydroxyl group is attached to the 3' carbon of the terminal deoxyribose; at the other end (5' end), a phosphate group is attached to the 5' carbon of the terminal de
oxyribose.
- DNA polymerase can only elongate strands in the 5' ® 3' direction.
The problem of antiparallel DNA strands is solved by the continuous synthesis of one strand (leading strand) and discontinuous synthesis of the complementary strand (lagging strand).
Leading strand = The DNA strand which is synthesized as a single polymer in the 5' ® 3' direction towards the replication fork.
Lagging strand = The DNA strand that is discontinuously synthesized against the overall direction of replication.
- Lagging strand is produced as a series of short segments called Okazaki ftagments which are each synthesized in the 5' ® 3' direction.
- Okazaki fragments are 1000-2000 nucleotides long in bacteria and 100 to 200 nucleotides long in eukaryotes.
- The many fragments are ligated by DNA ligase, a linking enzyme that catalyzes the formation of a covalent bond between the 3' end of each new Okazaki fragment to the 5' end of the growing chain.
- Priming DNA Synthesis
Before new DNA strands can form, there must be small pre-existing primers to start the addition of new nucleotides.
Primer = Short RNA segment that is complementary to a DNA segment and that is necessary to begin DNA replication.
- Primers are short segments of RNA polymerized by an enzyme called primase.
- A portion of the parental DNA serves as a template for making the primer with a complementary base sequence that is about 10 nucleotides long in eukaryotes.
- Primer formation must precede DNA replication, because DNA polymerase can only add nucleotides to a polynucleotide that is already correctly base-paired with a-complementary strand.
Only one primer is necessary for replication of the leading strand, but many primers are required to replicate the lagging strand.
- An RNA primer must initiate the synthesis of each Okazaki fragment.
- The many Okazaki fragments are ligated in two steps to produce a continuous DNA strand:
- DNA polymerase removes the RNA primer and replaces it with DNA.
- DNA ligase catalyzes the linkage between the 3' end of each new Okazaki fragment to the 5' end of the growing chain.
Back to top
V. Enzymes proofread DNA during its replication and repair damage to existing DNA.
DNA replication is highly accurate, but this accuracy is not solely the result of base-pairing specificity.
- Initial pairing errors occur at a frequency of about one in ten thousand, while errors in a complete DNA molecule are only about one in one billion.
- DNA can be repaired as it is being synthesized (e.g. mismatch repair) or after accidental changes in existing DNA (e.g. excision repair).
Mismatch repair, corrects mistakes when DNA is synthesized.
- In bacteria, DNA polymerase proofreads each newly added nucleotide against its template. If polymerase detects an incorrectly paired nucleotide, the enzyme removes and replaces it before continuing with synthesis.
- In eukaryotes, additional proteins as well as polymerase participate in mismatch repair. A hereditary defect in one of these proteins has been associated with a form of colon cancer. Apparently, DNA errors accumulate in the absence of ade
quate proofreading.
Excision repair, corrects accidental changes that occur in existing DNA.
- Accidental changes in DNA can result from exposure to reactive chemicals, radioactivity, X-rays and ultraviolet light.
- There are more than fifty different types of DNA repair enzymes that repair damage. For example, in excision repair:
Þ The damaged segment is excised by one repair enzyme and the remaining gap is filled in by base-pairing nucleotides with the undamaged strand.
Þ DNA polymerase and DNA ligase are enzymes that catalyze the filling-in process.
Course Pages maintained by
Dr. Graeme Lindbeck .