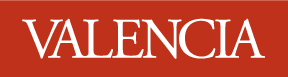
BSC 1010C
Fundamentals of Biology I
Mitosis
Outline
- Cell division functions in reproduction, growth, and repair
- Bacteria reproduce by binary fission
- The genome of a eukaryotic cell is organized into multiple
chromosomes
- Mitosis alternates with interphase in the cell cycle
- The Stages of Mitotic Cell Division
- The mitotic spindle distributes chromosomes to daughter cells
- Cytokinesis divides the cytoplasm
- External and internal cues control cell division
- Cyclical changes in regulatory proteins function as a mitotic
clock
- Cancer cells escape from the controls on cell division
The ability to reproduce distinguishes living organisms from nonliving objects-
this ability has a cellular basis.
"Where a cell exists, there must have been a preexisting cell, just as the
animal arises only from an animal and the plant only from a plant." "Omnis
cellula e cellula" or "All cells from cells." - Rudolf Virchow (1855).
Back to top
I. Cell division functions in reproduction, growth, and repair
The perpetuation of life is based on the reproduction of cells or cell division.
- In unicellular organisms, the division of one cell to form two reproduces
an entire organism (e.g. Amoeba).
- In multicellular organisms, cell division allows:
Þ Growth and development from the fertilized
egg
Þ Replacement of damaged or dead cells.
Cell division is a complex process that faithfully passes along the genome
from one generation of cells to the next. A dividing cell:
- Precisely replicates its DNA.
- Equally distributes the DNA to opposite ends of the cell.
- Separates into two identical daughter cells
Genome = Total endowment of DNA unique to each species.
Back to top
II. Bacteria reproduce by binary fission
Prokaryotes are smaller and simpler than eukaryotes. Prokaryotes:
- Contain most genes in a single circular chromosome composed of a double-stranded
DNA molecule and associated proteins.
- Contain only about 1/1000 the DNA of eukaryotes, but prokaryotic chromosomes
still contain a large amount of DNA relative to the small prokaryotic cell.
Consequently, bacterial chromosomes are highly folded and packed within the
cell.
Prokaryotes reproduce by binary fission, a process during which bacteria
replicate their chromosomes and equally distribute copies between the two daughter
cells.
- The chromosome is replicated; each copy remains attached to the plasma membrane
at adjacent sites.
- Between the attachment sites the membrane grows and separates the two copies
of the chromosome.
- The bacterium grows to about twice its initial size, and the plasma membrane
pinches inward.
- A cell wall forms across the bacterium between the two chromosomes, dividing
the original cell into two daughter cells.
Back to top
III. The genome of a eukaryotic cell is organized into multiple chromosomes
Dividing eukaryotic cells replicate and distribute their tens of thousands
of genes - a process that is manageable because the genes are organized into
multiple chromosomes.
Chromosome = Threadlike structures in eukaryotic nuclei that are composed
of DNA and protein.
- Each species has a characteristic chromosome number. (Human somatic cells
have 46.)
- Gametes (sperm and ova) contain half the number of chromosomes of somatic
cells. (Human gametes have 23.)
Chromatin, a DNA-protein complex, is organized into a long, thin fiber
that is folded and coiled to form the chromosome. Each chromosome thus contains:
- A long DNA molecule, with thousands of genes.
- Various proteins that maintain chromosomal structure and help control gene
activity.
Mitosis = (Mitos = thread) Nuclear division during which duplicated
chromosomes are evenly distributed into two daughter nuclei; results in two
daughter cells that are the genetic equivalent of the parent cell.
- Before mitosis, a cell copies its genome by duplicating every chromosome,
each of which forms two identical sister chromatids joined at a specialized
region called the centromere.
- During mitosis, sister chromatids are pulled apart forming two complete
chromosome sets, one at each end of the cell.
- Mitosis (division of the nucleus) may be followed by cytokinesis
(division of the cytoplasm).
Cytokinesis = Cytoplasmic division that forms two separate daughter
cells, each containing a single nucleus.
Back to top
IV. Mitosis alternates with interphase in the cell cycle
Cell cycle = Well-ordered sequence of events between the time a cell divides
to form two daughter cells and the time those daughter cells divide.
- Includes a doubling of a cell's cytoplasm, reproduction of cellular organelles,
precise duplication of DNA, mitosis and cytokinesis.
- Duration varies with cell type. Some cells divide each hour, others take
more than 24 hours.
- Nerve and muscle cells never or rarely divide once they are formed.
The cell cycle alternates between M phase, or dividing phase,
and Interphase, the nondividing phase:
- M phase, the shortest part of the cell cycle and the phase during
which the cell divides, includes:
- Mitosis - division of the nucleus.
- Cytokinesis - division of the cytoplasm.
- Interphase, the nondividing phase, includes most of a cell's growth and
metabolic activities.
Þ Is about 90% of the cell cycle.
Þ Is a period of intense biochemical activity
during which the cell grows and copies its chromosomes in preparation for
cell division.
Þ Consists of three periods:
- G1 phase - first growth phase. (G stands for "gap".)
- S phase - synthesis phase when DNA is synthesized as chromosomes are
duplicated. (S stands for "synthesis".)
- G2 phase - second growth phase.
Mitosis is unique to eukaryotes and may be an evolutionary adaptation for distributing
a large amount of genetic material.
- Details may vary, but overall process is similar in most eukaryotes.
- It is a reliable process with only one error per 100,000 cell divisions.
A. The Stages of Mitotic Cell Division
Mitosis and cytokinesis form a continuum, but for ease of description, mitosis
is usually divided into five stages: prophase, prometaphase, metaphase,
anaphase and telophase.
- G2 of Interphase: A G2 cell is characterized by:
- A well-defined nucleus bounded by a nuclear envelope.
- One or more nucleoli.
- Two centrosomes adjacent to the nucleus (formed earlier by replication
of a single centrosome).
- In animals, a pair of centrioles in each centrosome.
- In animals, a radial microtubular array (aster) around each
pair of centrioles
- Duplicated chromosomes that cannot be distinguished individually due
to loosely packed chromatin fibers. (Chromosomes were duplicated earlier
in S phase.)
- Prophase:
- In the nucleus:
- Nucleoli disappear.
- Chromatin fibers condense into discrete, observable chromosomes, composed
of two identical sister chromatids joined at the centromere.
- In the cytoplasm:
- Mitotic spindle forms. It is composed of microtubules between the
two centrosomes or microtubule-organizing centers.
- Centrosomes move apart, apparently propelled along the nuclear surface
by lengthening of the microtubute bundles between them.
- Prometaphase:
- During prometaphase:
- Nuclear envelo
pe fragments, which allows microtubules to interact with the highly
condensed chromosomes.
- Spindle fibers (bundles of microtubules) extend from each pole toward
the cell's equator.
- Each chromatid now has a specialized structure, the kinetochore,
located at the centromere region.
- Kinetochore microtubules become attached to the kinetochores
and put the chromosomes into agitated motion.
- Nonkinetochore microtubules radiate from each centrosome toward
the metaphase plate without attaching to chromosomes.
- Nonkinetochore microtubules radiating from one pole overlap with those
from the opposite pole.
- Metaphase:
- During metaphase:
- Centrosomes are positioned at opposite poles of the cell.
- Chromosomes move to the metaphase plate, the plane equidistant
between the spindle poles.
- Centromeres of all chromosomes are aligned on the metaphase plate.
- The long axis of each chromosome roughly at a right angle to the spindle
axis.
- Kinetochores of sister chromatids face opposite poles, so identical
chromatids are attached to kinetochore fibers radiating from opposite
ends of the parent cell.
- Entire structure formed by nonkinetochore microtubules plus kinetochore
microtubules is called the spindle.
- Anaphase:
- Anaphase is characterized by movement. It begins when paired centromeres
of each chromosome move apart.
- Sister chromatids split apart into separate chromosomes and move towards
opposite poles of the cell.
- Because kinetochore fibers are attached to the centromeres, the chromosomes
move centromere first in a "V" shape.
- Kinetochore microtubules shorten at the kinetochore end as chromosomes
approach the poles.
- Simultaneously, the poles of the cell move farther apart, elongating
the cell.
- At the end of anaphase, the two poles have identical collections of chromosomes.
- Telophase and Cytokinesis:
- During telophase:
- Nonkinetochore microtubules further elongate the cell.
- Daughter nuclei begin to form at the two poles.
- Nuclear envelopes form around the chromosomes from fragments of the
parent cell's nuclear envelope and portions of the endomembrane system.
- Nucleoli reappear.
- Chromatin fiber of each chromosome uncoils and the chromosomes become
less distinct.
- By the end of telophase:
- Mitosis, the equal division of one nucleus into two genetically identical
nuclei, is complete.
- Cytokinesis has begun and the appearance of two separate daughter
cells occurs shortly after mitosis is completed.
Back to top
V. The mitotic spindle distributes chromosomes to daughter cells
During prophase, the mitotic spindle forms in the cytoplasm from microtubules
and associated proteins.
- Microtubules of the cytoskeleton are partially disassembled during spindle
formation.
Þ Spindle microtubules are aggregates
of two proteins, a - and b -tubulin.
Þ Spindle microtubules elongate by the adding
tubulin subunits at one end.
- Parallel microtubules form bundles called spindle fibers that are
visible under a light microscope; their assembly begins in the centrosome
or rnicrotubule organizing center.
- In animal cells, a pair of centrioles is in the center of the centrosome,
but there is evidence that they are not essential for cell division:
Þ If the centrioles of animal cells are
destroyed with a laser microbeam, spindles still form and function during
mitosis.
Þ Plant centrosomes lack centrioles.
- Interphase: The centrosome replicates to form two centrosomes located just
outside the nucleus.
- Prophase and Prometaphase: The two centrosomes move farther apart.
- Spindle microtubules radiate from the centrosomes, elongating at the
end away from their centrosome.
- Late Prometaphase: By the end of prometaphase, the two centrosomes are at
opposite poles and the chromosomes have moved to the cell's midline.
- Each chromatid of a replicated chromosome develops its own kinetochore,
a protein structure on the centromere.
Þ Some spindle microtubules attach
to the kinetochores and are called kinetochore microtubules.
Þ Some spindle microtubules extend from
the centrosomes and overlap with those radiating from the cell's opposite
pole. These are called polar or nonkinetochore microtubules.
- Kinetochore microtubules interact to: (1) arrange the chromosomes so
kinetochores face the poles and (2) align the chromosomes at the cell's
midline.
- The most stable arrangement occurs when sister kinetochores are attached
by microtubules to opposite spindle poles.
Þ Initially, kinetochore niicrotubules
from one pole may attach to a kinetochore, moving the chromosome toward
that pole. This movement is checked when microtubules from the opposite
pole attach to the chromosome's other ki netochore.
Þ The chromosome oscillates back and forth
until it stabilizes and aligns at the cell's midline.
Þ Microtubules can remain attached to
a kinetochore only if there is opposing tension from the other side.
It is this opposing tension that stabilizes the microtubule- kinetochore
connection and allows the proper alignment and movement of chromosomes
at the cell's midline.
- Metaphase: All the duplicated chromosomes align on the cell's midline, or
metaphase plate.
- Anaphase: The chromosome's centromeres split and the sister chromatids move
as separate chromosomes toward opposite ends of the cell.
- How do the kinetochore microtubtiles function in this poleward movement
of chromosomes? Based on experimental evidence, the current model is that:
- Kinetochore microtubutes shorten during anaphase by depolymerizing at
their kinetochore ends; pulling the chromosomes poleward.
- The mechanism of this interaction between kinetochores and microtubules
may involve microtubule-walking proteins similar to dynein that "walk"
a chromosome along the shortening microtubules.
- What is the functiotin of the nonkinetochore microtubules?
- Nonkinetochore tubules elongate the whole cell along the polar axis
during anaphase.
- These tubules overlap at the middle of the cell and slide past each
other away from the cell's equator, reducing the degree of overlap.
- It is hypothesized that dynein cross-bridges may form between overlapping
tubules to slide them past one another. Alternatively, motor molecules
may link the microtubules to other cytoskeletal elements to drive the
sliding.
- ATP provides the energy for this endergonic process.
- Telophase: At the end of anaphase, the duplicate sets of chromosomes are
clustered at opposite ends of the elongated parent cell.
- Nuclei reform during telophase.
- Cytokinesis usually divides the cell's cytoplasm during late telophase.
In some exceptional cases, mitosis is not followed by cytokinests (e.g.
certain slime molds form multinucleated masses called plasmodia).
Back to top
VI. Cytokinesis divides the cytoplasm
Cytokinesis, the process of cytoplasmic division, begins in telophase
and is different in animal and plant cells. In animal cells, cytokinesis occurs
as cleavage:
- First, a cleavage furrow forms as a shallow groove in the cell surface
near the old metaphase plate.
- A contractile ring of actin microfilaments forms on the cytoplasmic
side of the furrow; this ring contracts until it pinches the parent cell in
two.
- Finally, the remaining mitotic spindle breaks, and the two cells become
completely separate.
In plant cells, cytokinesis occurs by cell plate formation across the
parent cell's midline (old metaphase plate).
- Golgi-derived vesicles move along microtubules to the cell's center, where
they fuse into a disc-like cell plate.
- Additional vesicles fuse around the edge of the plate, expanding it laterally
until its membranes touch and fuse with the existing parent cell's plasma
membrane.
- A new cell wall forms as cellulose is deposited between the two membranes
of the cell plate.
Back to top
VII. External and internal cues control cell division
Normal growth, development and maintenance depend on the timing and rate of
mitosis. Various cell types differ in their pattern of cell division; for example:
- Human skin cells divide frequently.
- Liver cells only divide in appropriate situations, such as wound repair.
- Nerve, muscle and other specialized cells do not divide in mature humans.
Using tissue culture, researchers have identified some factors that influence
cell division:
- Contents of the culture medium.
- If essential nutrients are left out of the culture medium, cells will
not divide.
- Specific regulatory substances called growth factors are necessary
for some cultured mammalian cells to divide. For example:
Þ Binding of platelet-derived growth
factor (PDGF) to cell membrane receptors, stimulates cell division in
fibroblasts. This regulation probably occurs not only in cell culture,
but in the animal's body as well - a response that helps heal wounds.
Þ Other cell types may have membrane receptors
for different growth factors or for different combinations of several
growth factors.
- Cell density.
- Crowding inhibits cell division in a phenomenon called density-dependent
inhibition. Cultured cells stop dividing when they form a single layer
on the container's inner surface. If some cells are removed, those bordering
the open space divi de again until the vacancy is filled.
- Density-dependent inhibition is apparently a consequence of the fact
that:
Þ Quantities of nutrients and growth
regulators may be insufficient to support cell division, if cell density
is too high.
Þ For normal cell division, cells must
adhere to a substratum - such as the surface of a culture dish. Cells
will stop dividing if they become detached.
- Density-dependent inhibition probably occurs in the body's tissues as
well as in cell culture. Cancer cells are abnormal and do not exhibit
density-dependent inhibition.
- G1 phase of the cell cycle.
- Whether a cell is destined to divide is determined at the restriction
point which occurs late in the G1 phase of the cell cycle.
Þ If a cell is destined to divide,
it progresses beyond the restriction point into the S phase when DNA
synthesis begins, and then proceeds through cell division.
Þ If the cell is not destined to divide,
it may exit from the cell cycle at the restriction point and switch
to a nondividing state called the G0 phase.
- Most human body cells are in the G0 phase. Some most specialized
cells, such as nerve and muscle, stay in G0 and never divide.
(Recent experimental evidence suggests that it may be possible to reinitiate
cell division in some n erve cells. This holds out the possibility that
some day spinal cord injuries may be repairable.). Other cells, such as
liver cells, can be induced by environmental cues, such as injury, to
continue through the cell cycle and divide.
- Cell size.
- For actively dividing cells, the ratio of cytoplasmic volume to genome
size is the most important indicator of whether a cell will pass the restriction
point.
- Once a cell's volume-to-genome ratio reaches a critical threshold value,
it may pass the restriction point and copy its DNA. Thus, a cell must
add enough cytoplasm to attain a certain size, before DNA synthesis can
begin. This prevents daughter ce lls from becoming progressively smaller
with each cell cycle.
Back to top
VIII. Cyclical changes in regulatory proteins function as a mitotic clock
Once the cell passes the restriction point, it is destined to divide.
- The onset of S phase commits the cell to continue through G2
and M phases and divide.
- Biologists are only beginning to work out the switches that control the
precise sequence of events in cell division. We do know that this sequence
probably depends on the completion of each task before the cell can progress
to the next stage.
The ordered sequence of cell cycle events is synchronized by rhythmic changes
in the activity of regulatory proteins, some of which are protein kinases.
- Protein kinases are enzymes that catalyze the transfer of a phosphate group
from ATP to a target protein.
- Phosphorylation, in turn, induces a conformational change that either activates
or inactivates the target protein.
Cyclical changes in kinase activity are controlled by another class of regulatory
proteins called cyclins.
- These regulatory proteins are named cyclins, because their concentrations
change cyclically during the cell cycle.
- Protein kinases that regulate cell cycles are cyclin-dependent kinases
(or Cdks); they are active only when attached to a particular cyclin.
- Even though Cdk concentration stays the same throughout the cell cycle,
its activity changes in response to the changes in cyclin concentration.
An example of a cyclin-dependent kinase is MPF (maturation promoting factor),
which controls the cell's progress from late interphase (G2) to mitosis.
- Active MPF phosphorylates chromatin proteins, causing chromosomes to condense
during prophase.
- During prometaphase, the nuclear envelope disperses when some of its membrane
proteins are phosphorylated.
Cyclin's rhythmic changes in concentration regulate MPF activity, and thus
act as a mitotic clock that regulates the sequential changes in a dividing cell.
- Cyclin is produced at a uniform rate throughout the cell cycle, and it accumulates
during interphase.
- Cyclin combines with Cdk to form active MPF, so as cyclin concentration
rises and falls, the amount of active MPF changes in a similar way.
- Active MPF activates proteins that participate in mitosis and initiates
prophase.
- Near the end of mitosis, cyclin is destroyed by an enzyme that is activated
by MPF.
- The destruction of cyclin causes the decline in active MPF at the end of
mitosis. Since MPF is activated by cyclin, it is indirectly responsible for
its own decline.
- Continuing cyclin synthesis raises the concentration again during interphase.
This newly synthesized cyclin binds to Cdk to form active MPF, and mitosis
begins again.
Rhythmic changes in different cyclin-Cdk complexes regulate other cell cycle
stages.
To summarize the control of the cell cycle:
- The G1 phase of the cycle is the most variable phase in duration
and in the variety of external and internal controls over cell division.
- Nutritional status, growth factors, cell density, and developmental state
of the cell affect the length of G1 and whether the cell will pass
the restriction point and divide.
- A cell that does not pass the restriction point will enter the G0
phase as a nondividing cell.
- If conditions favor cell division, the cell will proceed through the restriction
point. When the cell acquires enough cytoplasm to reach the threshold volume-to-genome
ratio, the cell will be irreversibly committed to divide.
- Rhythmic changes in cyclin concentration activate protein kinases at key
regulatory points in the cell cycle, controlling the sequence of events during
cell division.
Back to top
IX. Cancer cells escape from the controls on cell division
Cancer cells do not respond normally to controls on cell division. They divide
excessively, invade other tissues and, if unchecked, can kill the whole organism.
- Cancer cells in culture do not stop growing in response to cell density
(density-dependent inhibition); they continue to grow until nutrients in the
medium are exhausted.
- Cancer cells that stop dividing do so at random points in the cycle instead
of at the restriction point in G1.
- Cancer cells in culture are immortal in that they continue to divide indefinitely,
as long as nutrients are available. Normal mammalian cells in culture divide
only about 20 to 50 times before they stop.
Cells in tissue culture that have lost the normal controls on growth are said
to be transformed.
Transformation = The conversion of eukaryotic cells in tissue culture
to a condition of unregulated growth. The immune system normally destroys abnormal
cells that have converted from normal to cancer cells.
- If abnormal cells evade destruction, they may proliferate to form a tumor,
an unregulated growing mass of cells within otherwise normal tissue.
- If the cells remain at this original site, the mass is benign and
can be completely removed by surgery.
- A tumor is malignant if the cells have the ability to spread to other
parts of the body. Only a malignant tumor is said to be cancer.
Besides having anomalous cell cycles, malignant cells are abnormal in other
ways. For example, they may have:
- Unusual numbers of chromosomes.
- Aberrant metabolism.
- Lost attachments to neighboring cells and substratum - usually a consequence
of abnormal cell surface changes.
Detached cancer cells may spread into other tissues surrounding the original
tumor and may even enter the blood and lymph vessels of the circulatory system.
- These circulating cells can invade other parts of the body and proliferate
to form more tumors.
- This spread of cancer cells beyond their original sites is called metastasis.
- If a tumor metastasizes, it is usually treated with radiation and chemotherapy,
which is especially harmful to actively dividing cells.
Researchers are beginning to understand how a normal cell is transformed into
a cancerous one. In most cases, it is probably caused by an alteration of genes
that control cell division.
- Michael Bishop and Harold Varmus won the Nobel Prize for demonstrating that
genetic alterations can cause cancer.
- Knowledge of how changes in the genome lead to the heritable abnormalities
of cancer cells is still rudimentary and depends upon our increased understanding
of how normal cells work.
Course Pages maintained by
Dr. Graeme Lindbeck
.