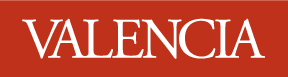
BSC 1010C
General Biology I
Dr.
Graeme Lindbeck
glindbeck@valenciacollege.edu

An Introduction to Metabolism
Outline
- The chemistry of life is organized into metabolic pathways
- Organisms transform energy
- The energy transformations of life are subject to two laws
of thermodynamics
- Organisms live at the expense of free energy
- Free Energy: A Criterion for Spontaneous Chance
- Free Energy and Equilibrium
- Free Energy and Metabolism
- ATP powers cellular work by coupling exergonic to endegonic
reactions
- The Structure and Hydrolysis of ATP
- How ATP Performs Work
- The Regeneration of ATP
- Enzymes speed up metabolic reactions by lowering energy barriers
- Enzymes are substrate-specific
- The active site is an enzyme's catalytic center
- A cell's chemical and physical environment affects enzyme
activity
- Effects of Temperature and pH
- Cofactors
- Enzyme Inhibitors
- Allosteric Regulation
- Cooperativity
- Metabolic order emerges from the cell's regulatory svstems
and structural organization
- Feedback Inhibition
- Structural Order and Metabolism
Back to top
I. The chemistry of life is organized into metabolic pathways
Metabolism = Totality of an organism's chemical processes.
- Property emerging from specific molecular interactions within the cell.
- Concerned with managing cellular resources: material and energy.
Metabolic reactions are organized into pathways that are orderly series of
enzymatically controlled reactions. Metabolic pathways are generally
of two types:
Catabolic pathways = Metabolic pathways which release energy by
breaking down complex molecules to simpler compounds. (e.g. Cellular respiration
which degrades glucose to carbon dioxide and water; provides energy for cellular
work.)
Anabolic pathways = Metabolic pathways which consume energy to
build complicated molecules from simpler ones. (e.g. Photosynthesis which synthesizes
glucose from CO2 and H2O; any synthesis of a macromolecule
from its monomers.)
Metabolic reactions may be coupled, so that energy released from a catabolic
reaction can be used to drive an anabolic one.
Back to top
II. Organisms transform energy
Energy = Capacity to do work.
Kinetic energy = Energy in the process of doing work (energy of motion).
For example:
- Heat (thermal energy) is kinetic energy expressed in random movement of
molecules.
- Light energy from the sun is kinetic energy which powers photosynthesis.
Potential energy = Energy that matter possesses because of its location
or arrangement (energy of position). For example:
- In the earth's gravitational field, an object on a hill or water behind
a dam have potential energy.
- Chemical energy is potential energy stored in molecules because of the arrangement
of nuclei and electrons in its atoms.
Energy can be transformed from one form to another. For example:
- Kinetic energy of sunlight can be transformed into the potential energy
of chemical bonds during photosynthesis.
- Potential energy in the chemical bonds of gasoline can be transformed into
kinetic mechanical energy which pushes the pistons of an engine.
Back to top
III. The energy transformations of life are subject to two
laws of thermodynamics
Thermodynamics = Study of energy transformations.
First Law of Thermodynamics = Energy can be transferred and transformed,
but it cannot be created or destroyed (energy of the universe is constant).
Second Law of Thermodynamics = Every energy transfer or transformation
makes the universe more disordered (every process increases the entropy of the
universe).
Entropy = Quantitative measure of disorder that is proportional to randomness
(designated by the letter S).
Closed system = Collection of matter under study which is isolated from
its surroundings.
Open system = System in which energy can be transferred between
the system and its surroundings.
The entropy of a system may decrease, but the entropy of the system plus its
surroundings must always increase. Highly ordered living organisms do not violate
the second law because they are open systems. For example, animals:
- Maintain highly ordered structure at the expense of increased entropy of
their surroundings.
- Take in complex high energy molecules as food and extract chemical energy
to create and maintain order.
- Return to the surroundings simpler low energy molecules (CO2
and water) and heat.
Energy can be transformed, but part of it is dissipated as heat which is largely
unavailable to do work. Heat energy can perform work if there is a heat gradient
resulting in heat flow from warmer to cooler.
Combining the first and second laws; the quantity of energy in the universe
is constants but its quality is not.
Back to top
IV. Organisms live at the expense of free energy
- Free Energy: A Criterion For Spontaneous Change
Not all of a system's energy is available to do work. The amount of energy
that is available to do work is described by the concept of free energy.
Free energy (G) is related to the system's total energy (H) and its entropy
(S) in the following way:
where:
G = Gibbs free energy (energy available to do work)
H = enthalpy or total energy
T = temperature in oK
S = entropy
Free energy (G) = Portion of a system's energy available to do work;
is the difference between the total energy (enthalpy) and the energy not available
for doing work (TS).
The maximum amount of useable energy that can be harvested from a particular
reaction is the system's free energy change from the initial to the final
state. This change in free energy (DG) is given
by the Gibbs-Helmholtz equation at constant temperature and pressure:
where:
DG = change in free energy
DH = change in total energy (enthalpy)
DS = change in entropy
T = absolute temperature in oK (which is oC + 273)
Significance of Free Energy:
- Indicates the maximum amount of a system's energy which is available to
do work.
- Indicates whether a reaction will occur spontaneously or not.
- A spontaneous reaction is one that will occur without additional
energy.
- In a spontaneous process, DG or free energy
of a system decreases (DG<0).
- A decrease in enthalpy (-DH) and an increase
in entropy (+DS) reduces the free energy
of a system and contributes to the spontaneity of a process.
- A higher temperature enhances the effect of an entropy change. Greater
kinetic energy of molecules tends to disrupt order as the chances for
random collisions increase.
- When enthalpy and entropy changes in a system have an opposite effect
on free energy, temperature may determine whether the reaction will
be spontaneous or not (e.g. protein denaturation by increased temperature).
- High energy systems, including high energy chemical systems, are unstable
and tend to change to a more stable state with a lower free energy.
- Free Energy and Equilibrium
There is a relationship between chemical equilibrium and the free energy
change (DG) of a reaction:
- As a reaction approaches equilibrium, the free energy of the system decreases
(spontaneous and exergonic reaction).
- When a reaction is pushed away from equilibrium, the free energy of system
increases (non-spontaneous and endergonic reaction).
- When a reaction reaches equilibrium, DG = 0,
because there is no net change in the system.
Metabolic Disequilibrium: Since many metabolic reactions are reversible,
they have the potential to reach equilibrium.
- At equilibrium, DG = 0, so the system can do
no work.
- Metabolic disequilibrium is a necessity of life; a cell at equilibrium
is dead.
- In the cell, these potentially reversible reactions are pulled forward
away from equilibrium, because the products of some reactions become reactants
for tile next reaction in the metabolic pathway.
- For example, during cellular respiration a steady supply of high energy
reactants such as glucose and removal of low energy products such as CO2
and H2O, maintain the disequilibrium necessary for respiration
to proceed.
- Free Energy and Metabolism
Reactions can be classified based upon their free energy changes:
Exergonic reaction = A reaction that proceeds with a net loss of free
energy.
Endergonic reaction = An energy-requiring reaction that proceeds with
a net gain of free energy; a reaction that absorbs free energy from its surroundings.
If a chemical process is exergonic, the reverse process must be endergonic.
For example:
- For each mole of glucose oxidized in the exergonic process of cellular
respiration, 2870 kJ are released (DG = -2870
kJ/mol or -686 kcal/mol).
- To produce a mole of glucose, the endergonic process of photosynthesis
requires an energy input of 2870 kJ (DG = +2870
kJ/mol or +686 kcal/mol).
In cellular metabolism, endergonic reactions are driven by coupling them
to reactions with a greater negative free energy (exergonic). ATP plays a
critical role in this energy coupling.
Back to top
V. ATP powers cellular work by coupling exergonic to endergonic
reactions
ATP is the immediate source of energy that drives most cellular work, which
includes:
- Mechanical work such as beating of cilia, muscle contraction, cytoplasmic
flow, and chromosome movement during mitosis and meiosis.
- Transport work such as pumping substances across membranes.
- Chemical work such as the endergonic process of polymerization.
- The Structure and Hydrolysis of ATP
ATP (adenosine triphosphate) = Nucleotide with unstable phosphate
bonds that the cell hydrolyzes for energy to drive endergonic reactions. ATP
consists of:
- Adenine, a nitrogenous base.
- Ribose, a five-carbon sugar.
- Chain of three phosphate groups.
Unstable bonds between the phosphate groups can be hydrolyzed in an exergonic
reaction that releases energy.
When the terminal phosphate bond is hydrolyzed, a phosphate group is removed
producing ADP (adenosine diphosphate).
- Under standard conditions in the laboratory, this reaction releases kJ/mol
(-7.3 kcal/mol).
- In a living cell, this reaction releases -55 kJ/mol (-13 kcal/mol) - about
77% more than under standard conditions.
The terminal phosphate bonds of ATP are unstable, so:
- The products of the hydrolysis reaction are more stable than the reactant.
- Hydrolysis of the phosphate bonds is thus exergonic as the system shifts
to a more stable state.
- How ATP Performs Work
Exergonic hydrolysis of ATP is coupled with endergonic processes by transferring
a phosphate group to another molecule.
- Phosphate transfer is enzymatically controlled.
- The molecule acquiring the phosphate (phosphorylated or activated intermediate)
becomes more reactive.
For example, conversion of glutamic acid to glutamine:
Glu
glutamic acid |
+ |
NH3
ammonia |
® |
Gln
glutamine |
DG = +14.2 kJ/mol (+3.4 kcal/mol)
(endergonic) |
Two step process of energy coupling with ATP hydrolysis:
(1) Hydrolysis of ATP and phosphorylation of glutamic acid.
Glu + ATP |
® |
Glu-P + ADP |
unstable |
phosphorylated |
intermediate |
(2) Replacement of the phosphate with the reactant ammonia.
Overall DG:
Glu + NH3 |
® |
Gln |
DG = +14.2 kJ/mol |
ATP |
® |
ADP + Pi |
DG = - 31.0 kJ/mol |
|
|
|
Net DG = - 16.8 kJ/mol |
|
|
|
(Overall process is exergonic.) |
- The Regeneration of ATP
ATP is continually regenerated by the cell.
Process is rapid (107 molecules used and regenerated/sec/cell).
Reaction is endergonic.
ADP + Pi |
® |
ATP |
|
DG = + 31 kJ/mol (+7.3 kcal/mol) |
Energy to drive the endergonic regeneration of ATP comes from the exergonic
process of cellular respiration.
Back to top
VI. Enzymes speed up metabolic reactions by lowering energy
barriers
Free energy change indicates whether a reaction will occur spontaneously, but
does not give information about the speed of reaction.
- A chemical reaction will occur spontaneously if it releases free energy
(-DG), but it may occur too slowly to be effective
in living cells.
- Biochemical reactions require enzymes to speed up and control reaction
rates.
Catalyst = Chemical agent that accelerates a reaction without being
permanently changed in the process, so it can be used over and over.
Enzymes = Biological catalysts, which are usually proteins.
Before a reaction can occur, the reactants must absorb energy to break chemical
bonds. This initial energy investment is the activation energy.
Free energy of activation(Activation energy) = Amount of energy
that reactant molecules must absorb to start a reaction (EA).
Transition state = Unstable condition of reactant molecules that have
absorbed sufficient free energy to react.
Energy profile of an exergonic reaction:
- Reactants must absorb enough energy (EA) to reach the transition
state (uphill portion of the curve). Usually the absorption of thermal energy
from the surroundings is enough to break chemical bonds.
- Reaction occurs and energy is released as new bonds form (downhill portion
of the curve).
- DG for the overall reaction is the difference
in free energy between products and reactants. In an exergonic reaction the
free energy of the products is less than reactants.
Even though a reaction is energetically favorable, there must be an initial
investment of activation energy (EA).
The breakdown of biological macromolecules is exergonic. However, these molecules
react very slowly at cellular temperatures because they cannot absorb
enough thermal energy to reach transition state.
In order to make these molecules reactive when necessary. Cells use biological
catalysts called enzymes, which:
- Are usually proteins.
- Lower EA, so the transition state can be reached at cellular
temperatures.
- Do not change the nature of a reaction (DG), but
speed up a reaction that would have occurred anyway.
- Are very selective for which reaction they will catalyze.
Back to top
VII. Enzymes are substrate-specific
Enzymes are specific for a particular substrate, and that specificity
depends upon the enzyme's three-dimensional shape.
Substrate = The substance an enzyme acts on and makes more reactive.
An enzyme binds to its substrate and catalyzes its conversion to product. The
enzyme is released in original form.
Substrate + enzyme |
® |
enzyme-substrate complex |
® |
product + enzyme |
The substrate binds to the enzyme's active site.
Active site = Restricted region of an enzyme molecule which binds to
the substrate.
- Is usually a pocket or groove on the protein's surface.
- Formed with only a few of the enzyme's amino acids.
- Determines enzyme specificity which is based upon a compatible fit between
the shape of an enzyme's active site and the shape of the substrate.
- Changes its shape in response to the substrate.
Þ As substrate binds to the active site,
it induces the enzyme to change its shape.
Þ This brings its chemical groups into positions
that enhance their ability to interact with the substrate and catalyze the
reaction.
Induced fit = Change in the shape of an enzyme's active site, which
is induced by the substrate.
Back to top
VIII. The active site is an enzyme's catalytic center
The entire enzymatic cycle is quite rapid.
Steps in the Catalytic Cycle of Enzymes:
- Substrate binds to the active site forming an enzyme-substrate complex.
Substrate is held in the active site by weak interactions (e.g. hydrogen bonds
and ionic bonds).
- Induced fit of the active site around the substrate. Side chains
of a few amino acids in the active site catalyze the conversion of substrate
to product.
- Product departs active site and the enzyme emerges in its original form.
Since enzymes are used over and over, they can be effective in very small
amounts.
Enzymes lower activation energy and speed up reactions by several mechanisms:
- Active site can hold two or more reactants in the proper position so they
may react.
- Induced fit of the enzyme's active site may distort the substrate's chemical
bonds, so less thermal energy (lower DG) is needed
to break them during the reaction.
- Active site might provide a microenvironment conducive to a particular type
of reaction (e.g. localized regions of low pH caused by acidic side chains
on amino acids at the active site).
- Side chains of amino acids in the active site may participate directly in
the reaction.
The initial substrate concentration partly determines the rate of an enzyme
controlled reaction.
- The higher the substrate concentration, the faster the reaction - up to
a limit.
- If substrate concentration is high enough, the enzyme becomes saturated
with substrate. (The active sites of all enzymes molecules are engaged.)
- When an enzyme is saturated, the reaction rate depends upon how fast the
active sites can convert substrate to product.
- When enzyme is saturated, reaction rate may be increased by adding more
enzyme.
Back to top
IX. A cell's chemical and physical environment affects enzyme
activity
Each enzyme has optimal environmental conditions that favor the most active
enzyme conformation.
- Effects of Temperature and pH
Optimal temperature allows the greatest number of molecular collisions without
denaturing the enzyme.
- Enzyme reaction rate increases with increasing temperature. Kinetic energy
of reactant molecules increases with rising temperature, which increases
substrate collisions with active sites.
- Beyond the optimal temperature, reaction rate slows. The enzyme denatures
when increased thermal agitation of molecules disrupts weak bonds that stabilize
the active conformation.
- Optimal temperature range of most human enzymes is 35o- 40oC.
Optimal pH range for most enzymes is pH 6 - 8.
- Some enzymes operate best at more extremes of pH. For example, the digestive
enzyme, pepsin, found in the acid environment of the stomach has an optimal
pH of 2.
- Cofactors
Cofactors = Small nonprotein molecules that are required for proper
enzyme catalysis.
- May bind tightly to active site.
- May bind loosely to both active site and substrate.
- Some are inorganic (e.g. metal atoms of zinc, iron or copper).
- Some are organic and are called coenzymes (e.g. most vitamins).
- Enzyme Inhibitors
Certain chemicals can selectively inhibit enzyme activity
- Inhibition may be irreversible if the inhibitor attaches by covalent
bonds.
- Inhibition may be reversible if the inhibitor attaches by weak
bonds.
Competitive inhibitors = Chemicals that resemble an enzyme's normal
substrate and compete with it for the active site.
- Block active site from the substrate.
- If reversible, the effect of these inhibitors can be overcome by increased
substrate concentration.
Noncompetitive inhibitor = Enzyme inhibitors that do not enter the
enzyme's active site, but bind to another part of the enzyme molecule.
- Causes enzyme to change its shape so the active site cannot bind substrate.
- May act as metabolic poisons (e.g. DDT, many antibiotics).
- Selective enzyme inhibition is an essential mechanism in the cell for
regulating metabolic reactions.
- Allosteric Regulation
Allosteric site = Specific receptor site on some part of the enzyme
molecule other than the active site.
- Most enzymes with allosteric sites have two or more polypeptide chains,
each with its own active site. Allosteric sites are often located where
the subunits join.
- Allosteric enzymes have two conformations, one catalytically active and
the other inactive.
- Binding of an activator to an allosteric site stabilizes the active
conformation.
- Binding of an inhibitor (noncompetitive inhibitor) to an allosteric
site stabilizes the inactive conformation.
- Enzyme activity changes continually in response to changes in the relative
proportions of activators and inhibitors (e.g. ATP/ADP).
- Subunits may interact so that a single activator or inhibitor at one allosteric
site will affect the active sites of the other subunits.
- Cooperativity
Substrate molecules themselves may enhance enzyme activity.
Cooperativity = The phenomenon where substrate binding to the active
site of one subunit induces a conformational change that enhances substrate
binding at the active sites of the other subunits.
Back to top
X. Metabolic order emerges from the cell's regulatory systems
and structural organization
Metabolic pathways are regulated by controlling enzyme activity.
- Feedback Inhibition
Feedback inhibition = Regulation of a metabolic pathway by its end
product, which inhibits an enzyme within the pathway.
Prevents the cell from wasting chemical resources by synthesizing more product
than is necessary.
- Structural Order and Metabolism
Cellular structure orders and compartmentalizes metabolic pathways.
Some enzymes have fixed locations in the cell because they are incorporated
into a membrane.
Dissolved enzymes and their substrates may be localized within organelles
such as chloroplasts and mitochondria.
Course Pages maintained by
Dr. Graeme Lindbeck.